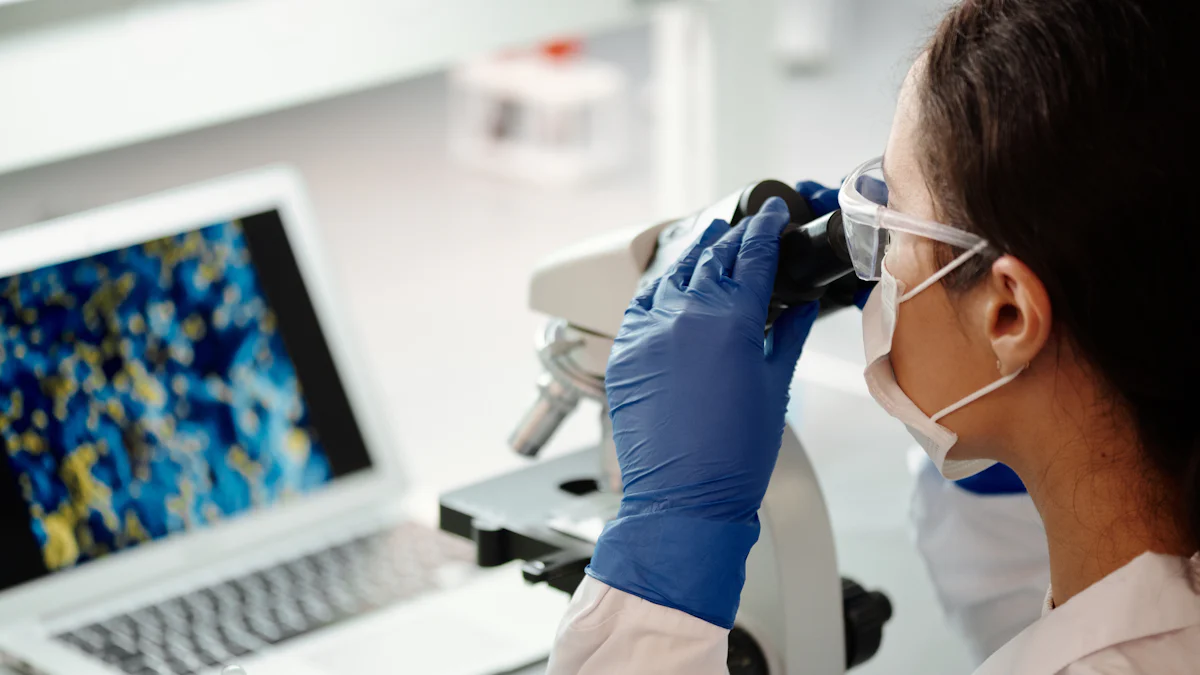
Applied materials epitaxy refers to the precise process of growing high-quality crystalline layers on substrates. This technique plays a pivotal role in enhancing material properties, such as electrical conductivity and structural integrity, making it indispensable for advanced technologies. Industries like semiconductors and optoelectronics rely heavily on this process to manufacture components with exceptional performance. For instance, epitaxy enables the production of defect-free layers essential for microchips and LEDs. The global epitaxy equipment market, valued at $1.7 billion in 2023, highlights its growing importance in modern manufacturing. Applied materials epitaxy trays further optimize this process by ensuring uniformity and precision during crystal growth.
Key Takeaways
- Applied materials epitaxy is essential for creating high-quality crystalline layers, crucial for advanced technologies like semiconductors and optoelectronics.
- The epitaxy process minimizes defects in materials, enhancing their electrical conductivity and structural integrity, which is vital for high-performance devices.
- Different epitaxy techniques, such as Molecular Beam Epitaxy (MBE) and Chemical Vapor Deposition (CVD), offer unique advantages for specific applications, from microchips to LEDs.
- Epitaxy supports the development of innovative materials for emerging technologies, including quantum computing, 5G, and sustainable energy solutions.
- Despite its benefits, epitaxy faces challenges like high equipment costs and technical complexity, which can limit accessibility for smaller manufacturers.
- Automation and AI are transforming the epitaxy process, improving precision and efficiency while reducing defect rates, making it more scalable for mass production.
- The future of epitaxy looks promising, with ongoing advancements expected to drive progress in various industries and enhance the performance of next-generation devices.
Understanding the Epitaxy Process in Applied Materials
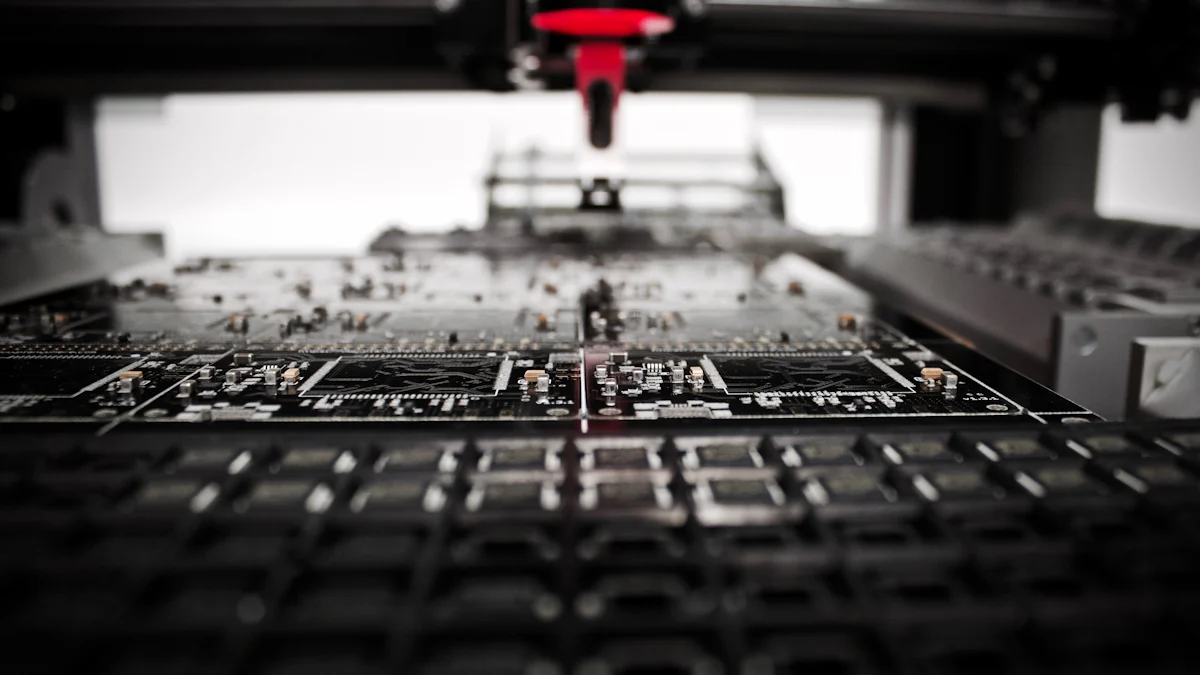
What Is Epitaxy in Material Science?
Epitaxy, a cornerstone of material science, refers to the process of growing a crystalline layer on a substrate where the crystal structure aligns with the underlying material. This alignment ensures the formation of high-quality layers with minimal defects. In semiconductor fabrication, epitaxy enables the creation of thin films with precise orientations, which are essential for electronic and photonic devices. By controlling the growth conditions, scientists can engineer materials with specific electrical, optical, or mechanical properties. This precision makes epitaxy indispensable in industries that demand advanced material performance.
Why Is Epitaxy Important for Material Quality?
Epitaxy plays a critical role in enhancing material quality. It allows materials to transmit electrons efficiently, which is vital for high-performance electronic devices. The process minimizes structural defects, ensuring the production of defect-free crystalline layers. These layers improve the reliability and functionality of components like microchips, LEDs, and solar cells. Additionally, epitaxy provides the foundation for creating materials with tailored properties, such as increased conductivity or enhanced optical performance. Without epitaxy, achieving the level of precision required in modern technologies would be nearly impossible.
How Does the Epitaxy Process Work?
The epitaxy process involves depositing a crystalline film onto a substrate under carefully controlled conditions. Factors like temperature, pressure, and gas flow must be precisely managed to ensure successful crystal growth. The process can be divided into several key stages:
The Role of Substrates in Epitaxy
Substrates serve as the foundation for epitaxial growth. Their crystal structure determines the alignment and quality of the grown layer. A substrate with a compatible lattice structure ensures seamless integration with the deposited material. For instance, in homoepitaxy, the substrate and the film share the same material, resulting in a perfect match. In heteroepitaxy, different materials are used, requiring meticulous control to minimize mismatches and defects.
Layer-by-Layer Crystal Growth Mechanism
Epitaxy relies on a layer-by-layer growth mechanism. Atoms or molecules are deposited onto the substrate surface, where they arrange themselves in a crystalline pattern. This process occurs one atomic layer at a time, allowing for precise control over the thickness and composition of the film. Advanced techniques, such as molecular beam epitaxy (MBE) and chemical vapor deposition (CVD), enable the deposition of ultra-thin layers with atomic-level accuracy. This meticulous approach ensures the production of high-quality materials for cutting-edge applications.
Types of Epitaxy Used in Applied Materials
Molecular Beam Epitaxy (MBE)
Molecular Beam Epitaxy (MBE) is a highly precise technique for growing crystalline layers. It operates in an ultra-high vacuum environment, where molecular or atomic beams are directed onto a heated substrate. This method allows for atomic-level control over the deposition process, making it ideal for creating advanced structures like quantum dots and quantum cascade lasers. MBE provides extensive in-situ monitoring options, enabling real-time observation of the growth process. Researchers often choose MBE for its ability to produce defect-free layers with exceptional uniformity. However, the high cost of equipment and operation limits its use to specialized applications.
Chemical Vapor Deposition (CVD)
Chemical Vapor Deposition (CVD) is a widely used epitaxy technique that relies on chemical reactions to deposit thin films. In this process, gaseous precursors react on the substrate surface to form a solid crystalline layer. CVD offers versatility, as it can deposit a broad range of materials by adjusting the precursor chemistry. This method is commonly employed in semiconductor manufacturing due to its scalability and efficiency. CVD enables the production of high-quality layers for devices like microchips and LEDs. Its ability to achieve precise control over film thickness and composition makes it a cornerstone of applied materials epitaxy.
Metalorganic Chemical Vapor Deposition (MOCVD)
Metalorganic Chemical Vapor Deposition (MOCVD) is a specialized form of CVD that uses metalorganic compounds as precursors. This technique dominates the III-V compound semiconductor epitaxy industry, particularly for materials like gallium arsenide (GaAs), gallium nitride (GaN), and indium phosphide (InP). MOCVD excels in precision and cost-effectiveness, making it the preferred choice for mass production. It supports epitaxial regrowth, allowing for complex device fabrication through etching and masking. Industries rely on MOCVD for manufacturing LEDs, laser diodes, and other optoelectronic components. Its ability to deliver high-quality layers at a lower cost than MBE underscores its importance in modern technology.
Liquid Phase Epitaxy (LPE)
Liquid Phase Epitaxy (LPE) represents one of the earliest and most straightforward methods for growing crystalline layers. This technique involves dissolving the material to be deposited in a liquid solvent at high temperatures. The substrate is then immersed in this solution, where the material crystallizes onto its surface as the solution cools. LPE offers a cost-effective approach for producing high-quality layers with minimal equipment requirements.
LPE excels in applications requiring thick epitaxial layers, such as power electronics and optoelectronic devices. Its simplicity makes it suitable for producing materials like gallium arsenide (GaAs) and gallium phosphide (GaP). However, LPE lacks the precision of more advanced techniques like Molecular Beam Epitaxy (MBE) or Metalorganic Chemical Vapor Deposition (MOCVD). Despite this limitation, industries continue to use LPE for specific applications where cost and simplicity outweigh the need for atomic-level control.
Homoepitaxy vs. Heteroepitaxy
Epitaxy can be categorized into two main types: homoepitaxy and heteroepitaxy. These classifications depend on the relationship between the substrate and the deposited material.
-
Homoepitaxy: This process involves growing a crystalline layer using the same material as the substrate. For example, depositing silicon on a silicon wafer exemplifies homoepitaxy. This method ensures perfect lattice matching, resulting in defect-free layers. Semiconductor manufacturers often use homoepitaxy to enhance the properties of existing materials without introducing mismatches.
-
Heteroepitaxy: In contrast, heteroepitaxy involves depositing a material different from the substrate. For instance, growing gallium nitride (GaN) on a sapphire substrate is a common example. Heteroepitaxy enables the creation of unique material combinations, unlocking new possibilities for advanced devices. However, lattice mismatches between the substrate and the film can lead to defects, requiring precise control during the growth process.
Both types play critical roles in applied materials epitaxy. Homoepitaxy ensures high-quality layers for applications demanding uniformity, while heteroepitaxy expands the range of achievable material properties. Together, they form the foundation for innovations in semiconductors, optoelectronics, and other advanced technologies.
Key Applications of Applied Materials Epitaxy
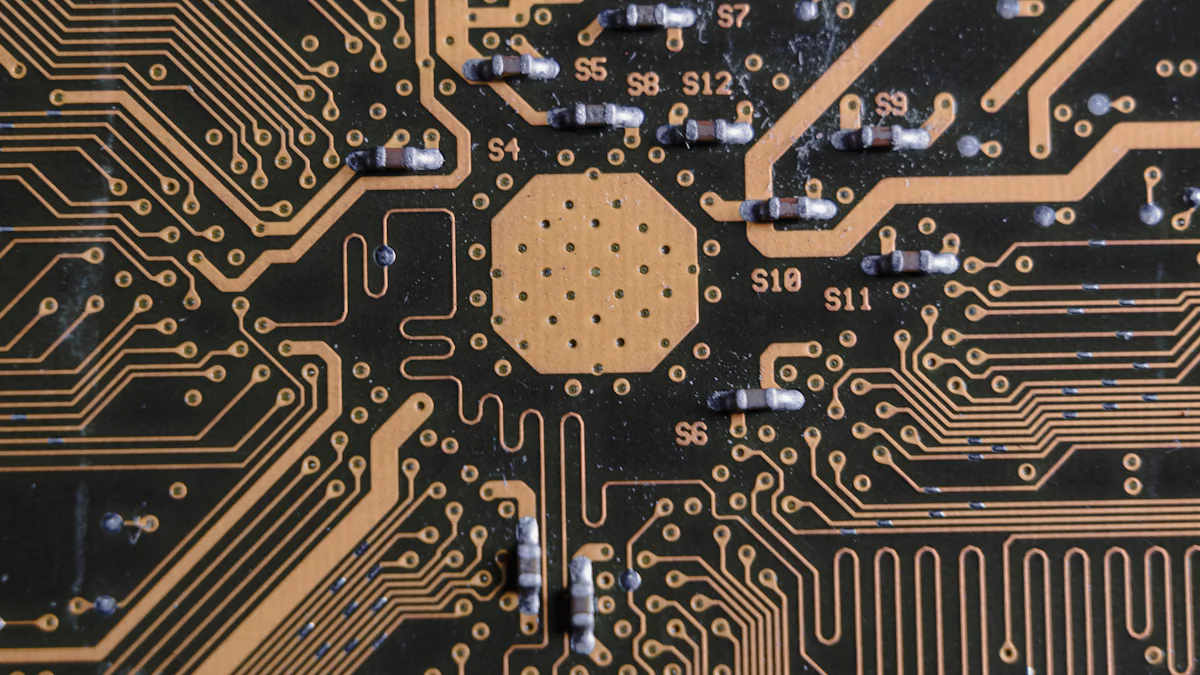
Semiconductor Industry
Role in Microchip Production
Applied materials epitaxy plays a vital role in microchip production. The process enables the creation of defect-free crystalline layers, which serve as the foundation for microchip components. These layers ensure optimal electrical conductivity and structural integrity, both of which are essential for high-performance microchips. Manufacturers rely on epitaxy to produce thin films with precise thickness and composition, allowing for the miniaturization of microchips without compromising their efficiency. This precision supports the development of faster and more reliable electronic devices, meeting the growing demand for advanced computing technologies.
Use in Transistors and Integrated Circuits
Epitaxy significantly enhances the performance of transistors and integrated circuits. By providing high-quality crystalline layers, it improves electron mobility within these components. This improvement results in faster switching speeds and reduced power consumption. In integrated circuits, epitaxial layers enable the seamless integration of multiple transistors, ensuring consistent performance across the entire chip. The ability to engineer materials with specific electrical properties through epitaxy has revolutionized the semiconductor industry, paving the way for innovations in consumer electronics, telecommunications, and data processing.
Optoelectronics
Applications in LED and Laser Manufacturing
In optoelectronics, applied materials epitaxy is indispensable for manufacturing LEDs and lasers. The process allows for the precise deposition of materials like gallium nitride (GaN) and gallium arsenide (GaAs), which are critical for these devices. Epitaxial growth ensures uniformity in the crystalline structure, resulting in LEDs with higher brightness and energy efficiency. For lasers, epitaxy enables the creation of layers with specific optical properties, ensuring consistent wavelength emission and improved performance. Industries leverage this technology to produce high-quality optoelectronic components for applications in displays, communication systems, and medical devices.
Use in Solar Cells and Photodetectors
Epitaxy also plays a crucial role in the development of solar cells and photodetectors. The process allows for the growth of thin films with enhanced light absorption and electrical conductivity. In solar cells, epitaxial layers improve energy conversion efficiency by minimizing defects that could hinder electron flow. Photodetectors benefit from epitaxy through the creation of materials with tailored optical properties, enabling the detection of specific wavelengths of light. These advancements contribute to the development of more efficient renewable energy solutions and high-performance imaging technologies.
Advanced Materials
Epitaxy in Quantum Computing Materials
Quantum computing relies heavily on advanced materials created through epitaxy. The process enables the growth of ultra-pure crystalline layers required for quantum bits (qubits). These layers exhibit exceptional coherence properties, which are essential for maintaining quantum states. Epitaxy allows researchers to engineer materials with precise atomic arrangements, facilitating the development of quantum devices with improved stability and performance. This capability positions epitaxy as a cornerstone in the quest to realize practical quantum computing technologies.
High-Performance Sensors and Emerging Technologies
Epitaxy supports the creation of high-performance sensors and other emerging technologies. The process enables the growth of materials with enhanced sensitivity and durability, which are critical for sensors used in medical diagnostics, environmental monitoring, and industrial automation. Additionally, epitaxy contributes to the development of advanced materials for applications in 5G communication, Internet of Things (IoT) devices, and sustainable energy solutions. By providing a platform for innovation, applied materials epitaxy continues to drive progress in cutting-edge technologies.
Advantages of Applied Materials Epitaxy
Precision and Scalability
Atomic-Level Control of Material Properties
Applied materials epitaxy enables atomic-level precision in controlling material properties. This process allows engineers to manipulate the electrical, optical, and mechanical characteristics of materials with unparalleled accuracy. By depositing atoms layer by layer, epitaxy ensures that the resulting crystalline structures meet exact specifications. This precision is critical for industries like semiconductors, where even minor deviations can compromise device performance. For example, in microchip production, epitaxy ensures uniform electron flow, which enhances processing speed and energy efficiency.
Expert Testimony:
Britannica, an authority in semiconductor technology, highlights that epitaxy is the only affordable method of high-quality crystal growth for many semiconductor materials. This underscores its importance in achieving precise material engineering at a reasonable cost.
Scalability for Mass Production
Epitaxy not only excels in precision but also supports scalability for mass production. Advanced epitaxial techniques, such as Chemical Vapor Deposition (CVD) and Metalorganic Chemical Vapor Deposition (MOCVD), allow manufacturers to produce large volumes of high-quality materials efficiently. These methods ensure consistent results across multiple production cycles, making them ideal for industries requiring high throughput. For instance, the optoelectronics sector relies on epitaxy to produce millions of LEDs and laser diodes annually. The scalability of epitaxy bridges the gap between laboratory innovation and industrial application, driving advancements in modern technology.
High-Quality Crystalline Layers
Defect-Free Material Growth
Epitaxy ensures the growth of defect-free crystalline layers, which form the foundation of high-performance devices. By aligning the crystal structure of the deposited layer with the substrate, epitaxy minimizes dislocations and other structural imperfections. This defect-free growth enhances the reliability and durability of components like transistors, LEDs, and solar cells. In semiconductor fabrication, defect-free layers improve electron mobility, resulting in faster and more efficient devices. The ability to produce flawless materials positions epitaxy as a cornerstone of advanced manufacturing.
Expert Testimony:
According to Britannica, epitaxy is used in the growth of semiconductor materials for forming layers and quantum wells in electronic and photonic devices. This capability highlights its role in producing defect-free structures essential for cutting-edge applications.
Enhanced Performance in Electronics and Optics
High-quality epitaxial layers significantly enhance the performance of electronic and optical devices. In electronics, these layers improve conductivity and reduce energy loss, enabling the development of faster and more efficient microchips. In optics, epitaxy allows for the creation of materials with tailored refractive indices, which optimize light emission and absorption. For example, epitaxial growth of gallium nitride (GaN) has revolutionized LED technology by increasing brightness and energy efficiency. The ability to engineer materials with superior properties ensures that epitaxy remains integral to technological progress.
Challenges of Applied Materials Epitaxy
High Costs of Equipment and Materials
Applied materials epitaxy demands advanced equipment and high-purity materials, which significantly increase production costs. The precision required for epitaxial growth necessitates specialized systems like Molecular Beam Epitaxy (MBE) and Metalorganic Chemical Vapor Deposition (MOCVD). These systems operate under ultra-high vacuum or controlled chemical environments, making them expensive to manufacture and maintain. Additionally, the raw materials used, such as gallium arsenide (GaAs) and indium phosphide (InP), often come with high price tags due to their rarity and purity requirements.
Industry Insight:
According to market analyses, epitaxy equipment has become indispensable in semiconductor and photonics manufacturing. However, the high initial investment and operational costs remain a barrier for smaller manufacturers.
The financial burden of these costs limits accessibility for many industries, particularly those operating on tight budgets. As a result, only large-scale manufacturers or research institutions can fully leverage the benefits of epitaxy, creating a gap in technological adoption.
Technical Complexity in Process Control
Epitaxy involves intricate processes that require precise control over multiple variables, including temperature, pressure, and gas flow. Even minor deviations can lead to defects in the crystalline layers, compromising the quality of the final product. For instance, heteroepitaxy, which involves growing a layer of material different from the substrate, often faces challenges like lattice mismatches. These mismatches can result in dislocations or strain, affecting the material’s performance.
Scientific Research Findings:
High-quality epitaxial growth of thin films, such as lithium niobate (LiNbO3), often struggles to balance material quality with growth rates. This highlights the technical challenges in achieving both precision and efficiency.
Engineers must continuously monitor and adjust the process to ensure optimal results. This level of complexity requires skilled personnel and advanced monitoring tools, further increasing operational costs. The steep learning curve associated with mastering epitaxial techniques also slows down the adoption of this technology in emerging markets.
Environmental and Energy Concerns
The epitaxy process consumes significant amounts of energy, particularly in techniques like MBE and CVD, which require high temperatures and vacuum conditions. This energy-intensive nature contributes to a larger carbon footprint, raising concerns about environmental sustainability. Additionally, the use of toxic gases and chemicals, such as arsine and phosphine, poses risks to both human health and the environment.
Environmental Perspective:
Epitaxy equipment manufacturers face increasing pressure to develop eco-friendly solutions. The industry must address the environmental impact of high energy consumption and hazardous waste generation.
Disposing of these hazardous materials safely adds another layer of complexity and cost to the process. As global industries move toward greener practices, the epitaxy sector must innovate to reduce its environmental impact while maintaining high-quality production standards.
The Future of Applied Materials Epitaxy
Innovations in Epitaxy Technology
Advances in Automation and AI
Automation and artificial intelligence (AI) are transforming the epitaxy process. Automated systems now monitor and adjust critical parameters such as temperature, pressure, and gas flow with unparalleled precision. This reduces human error and ensures consistent quality across production cycles. AI algorithms analyze vast amounts of data generated during epitaxial growth. These insights help optimize conditions for specific materials, improving efficiency and reducing waste.
For example, AI-driven models predict potential defects in real-time, allowing engineers to make immediate adjustments. This capability is particularly valuable in heteroepitaxy, where lattice mismatches often lead to structural imperfections. By leveraging automation and AI, manufacturers can achieve higher throughput while maintaining the stringent quality standards required for advanced applications.
Industry Insight:
A recent study highlights how AI integration in epitaxy has reduced defect rates by up to 30%, showcasing its potential to revolutionize material science.
Development of New Epitaxial Materials
The development of new epitaxial materials is expanding the possibilities for applied materials epitaxy. Researchers are exploring novel compounds like gallium oxide (Ga2O3) and aluminum nitride (AlN) for their unique properties. These materials offer higher thermal stability and improved electrical performance, making them ideal for next-generation devices.
In quantum computing, epitaxial growth of ultra-pure silicon carbide (SiC) and diamond substrates is enabling the creation of qubits with exceptional coherence times. Similarly, advancements in lithium niobate epitaxy are addressing historical challenges in achieving high-quality growth at practical rates. These breakthroughs are paving the way for innovations in photonics, power electronics, and other emerging fields.
Expanding Applications in Emerging Technologies
Role in 5G, IoT, and Sustainable Energy Solutions
Applied materials epitaxy is playing a pivotal role in the advancement of 5G, the Internet of Things (IoT), and sustainable energy solutions. In 5G technology, epitaxial layers enhance the performance of high-frequency transistors, enabling faster data transmission and improved network reliability. IoT devices benefit from the miniaturization and energy efficiency made possible by epitaxial growth, which supports the integration of sensors and processors in compact designs.
In sustainable energy, epitaxy is driving progress in solar cell technology. High-quality epitaxial layers improve light absorption and energy conversion efficiency, making renewable energy more accessible and cost-effective. Additionally, epitaxial materials are being used to develop advanced batteries and fuel cells, further supporting the global transition to cleaner energy sources.
Future Outlook:
Experts predict that the demand for epitaxial materials in 5G and IoT applications will grow by over 20% annually, underscoring their importance in shaping the future of technology.
Applied materials epitaxy serves as a cornerstone for advancing modern technologies. Its ability to create high-quality crystalline layers has revolutionized industries such as semiconductors, optoelectronics, and quantum computing. By enabling precise material engineering, it supports the development of faster, more efficient devices. The process continues to drive innovation, addressing challenges like scalability and environmental impact. As industries evolve, epitaxy remains essential for unlocking new possibilities in emerging fields. Continued advancements will ensure its pivotal role in shaping the future of technology.
FAQ
What is epitaxy, and why is it important?
Epitaxy refers to the process of growing crystalline layers on a substrate where the crystal structure aligns with the underlying material. This alignment ensures high-quality layers with minimal defects. It plays a vital role in semiconductor production by enabling controlled crystal growth, which enhances material properties like electrical conductivity and structural integrity. These improvements are essential for creating advanced electronic and photonic devices.
How does epitaxy contribute to semiconductor manufacturing?
Epitaxy enables the formation of defect-free crystalline thin films, which serve as the foundation for semiconductor wafers. These films allow materials to transmit electrons efficiently, improving the performance of microchips, transistors, and integrated circuits. By providing precise control over material properties, epitaxy supports the miniaturization and optimization of semiconductor components.
What are the main types of epitaxy used in applied materials?
The primary types of epitaxy include:
- Molecular Beam Epitaxy (MBE): Offers atomic-level precision in ultra-high vacuum environments.
- Chemical Vapor Deposition (CVD): Relies on chemical reactions to deposit thin films.
- Metalorganic Chemical Vapor Deposition (MOCVD): A specialized form of CVD used for III-V compound semiconductors.
- Liquid Phase Epitaxy (LPE): A cost-effective method for producing thick crystalline layers.
- Homoepitaxy and Heteroepitaxy: Differ based on whether the substrate and film materials are the same or different.
Each method serves specific applications, from microchip production to optoelectronic device fabrication.
What industries benefit most from epitaxy?
Epitaxy is indispensable in industries such as:
- Semiconductors: For microchips, transistors, and integrated circuits.
- Optoelectronics: For LEDs, lasers, and solar cells.
- Advanced Materials: For quantum computing, high-performance sensors, and emerging technologies like 5G and IoT.
Its ability to create high-quality crystalline layers makes it a cornerstone of modern technology.
How does epitaxy improve optoelectronic devices?
Epitaxy allows for the precise deposition of materials like gallium nitride (GaN) and gallium arsenide (GaAs), which are critical for optoelectronic devices. This process ensures uniformity in the crystalline structure, resulting in brighter, more energy-efficient LEDs and lasers with consistent wavelength emission. It also enhances the light absorption and energy conversion efficiency of solar cells and photodetectors.
What challenges does epitaxy face?
Epitaxy faces several challenges, including:
- High Costs: Advanced equipment and high-purity materials increase production expenses.
- Technical Complexity: Precise control over variables like temperature and pressure is required to avoid defects.
- Environmental Concerns: The process consumes significant energy and involves hazardous chemicals, raising sustainability issues.
Overcoming these challenges requires innovation in equipment design and process optimization.
How does automation and AI impact epitaxy?
Automation and artificial intelligence (AI) are revolutionizing epitaxy by improving precision and efficiency. Automated systems monitor and adjust parameters like temperature and gas flow, reducing human error. AI algorithms analyze data in real-time to predict and prevent defects. These advancements enhance scalability and ensure consistent quality, making epitaxy more accessible for mass production.
What role does epitaxy play in quantum computing?
Epitaxy enables the growth of ultra-pure crystalline layers required for quantum bits (qubits). These layers exhibit exceptional coherence properties, which are essential for maintaining quantum states. By engineering materials with precise atomic arrangements, epitaxy supports the development of stable and high-performing quantum devices, driving progress in quantum computing technologies.
Can epitaxy contribute to sustainable energy solutions?
Yes, epitaxy plays a significant role in advancing sustainable energy technologies. It improves the efficiency of solar cells by creating defect-free layers that enhance light absorption and energy conversion. Additionally, epitaxial materials are being used to develop advanced batteries and fuel cells, supporting the global shift toward cleaner energy sources.
What is the future of epitaxy in emerging technologies?
Epitaxy will continue to drive innovation in emerging fields like 5G, IoT, and renewable energy. Its ability to create high-quality materials with tailored properties ensures its relevance in next-generation devices. Advances in automation, AI, and new epitaxial materials will further expand its applications, solidifying its role as a cornerstone of technological progress.