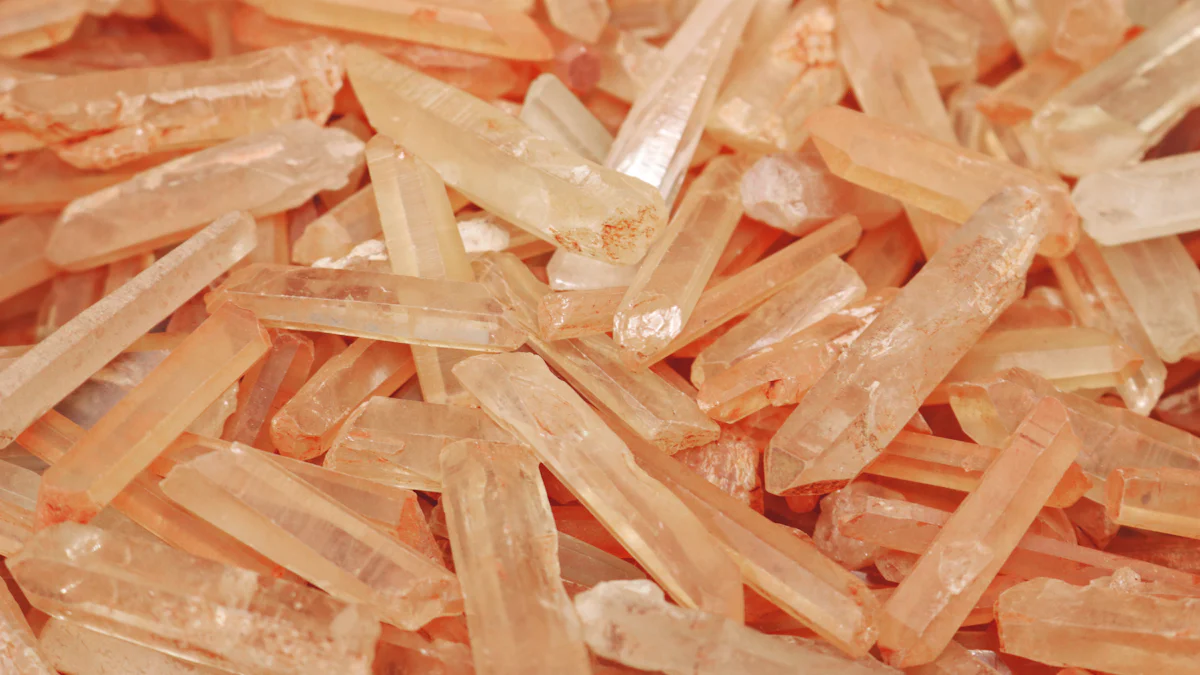
Epitaxy refers to the precise process of growing a crystalline layer on a substrate, ensuring the new layer aligns perfectly with the underlying structure. This technique plays a pivotal role in material science, especially in semiconductors and optoelectronics. By enabling the creation of high-quality crystalline layers, applied materials epitaxy enhances the performance of devices like transistors, LEDs, and solar cells. The demand for applied materials epitaxy continues to grow, driven by its ability to refine material properties and support advanced technologies. From applied materials epitaxy trays to semiconductor wafers and optoelectronic devices, epitaxy remains indispensable in modern innovation.
Key Takeaways
- Epitaxy is essential for creating high-quality crystalline layers, which enhance the performance of devices like transistors, LEDs, and solar cells.
- There are several types of epitaxy, including homoepitaxy and heteroepitaxy, each serving unique applications in semiconductor and optoelectronic manufacturing.
- Molecular Beam Epitaxy (MBE) and Chemical Vapor Deposition (CVD) are advanced techniques that offer precision and scalability, crucial for producing high-performance materials.
- Epitaxy allows for the customization of material properties, enabling innovations in fields like quantum computing and nanotechnology.
- Despite its advantages, epitaxy faces challenges such as high costs and complexity, which can limit its accessibility for smaller operations.
- The ability to create defect-free layers through epitaxy is vital for the reliability and efficiency of modern electronic devices.
- Epitaxy’s role in renewable energy, particularly in improving solar cell efficiency, highlights its importance in advancing sustainable technologies.
What Is Epitaxy?
Epitaxy is a fascinating process that forms the backbone of many advanced technologies. At its core, epitaxy involves growing a crystalline layer on a substrate, ensuring the new layer aligns perfectly with the atomic structure beneath it. This precise alignment is what makes epitaxy so valuable in creating high-quality materials for cutting-edge applications.
Definition of Epitaxy
Epitaxy refers to the controlled growth of a crystalline film on a substrate. The term originates from the Greek words epi (meaning “upon”) and taxis (meaning “arrangement”). This process ensures that the atoms in the new layer follow the same structural pattern as the substrate. By doing so, epitaxy minimizes defects and enhances the material’s overall quality. Scientists and engineers rely on epitaxy to create materials with specific properties, such as improved electrical conductivity or tailored optical characteristics.
Importance of Epitaxy in Applied Materials
Role in Material Science and Technology
Epitaxy plays a critical role in advancing material science. It allows researchers to design materials with exceptional precision, enabling innovations in fields like nanotechnology, renewable energy, and optoelectronics. For instance, epitaxy helps create monolayer and multilayer films, which are essential for studying surface interactions and developing new materials. This process also refines the structure of materials, ensuring they transmit electrons more efficiently and perform better in various applications.
Moreover, epitaxy supports the development of multifunctional devices. By layering materials with different properties, engineers can design devices that perform multiple tasks simultaneously. This capability is crucial for creating compact, high-performance technologies.
Connection to Semiconductors and Optoelectronics
In semiconductor fabrication, epitaxy is indispensable. It provides a flawless crystalline foundation for building semiconductor devices, such as transistors and integrated circuits. This foundation ensures that the devices operate with high efficiency and reliability. Additionally, epitaxy enables the deposition of films with engineered electrical properties, which is vital for enhancing device performance.
Optoelectronics also benefits significantly from epitaxy. The process is used to create materials for LEDs, laser diodes, and solar cells. These devices rely on epitaxial layers to achieve the precise optical and electrical properties needed for optimal performance. For example, epitaxy allows the creation of materials that emit light at specific wavelengths, making it possible to produce highly efficient LEDs.
Epitaxy’s importance extends to the tools and equipment used in these industries. For instance, applied materials epitaxy trays play a key role in supporting the growth of crystalline layers during the manufacturing process. These trays ensure uniformity and precision, which are essential for producing high-quality materials.
Types of Epitaxy
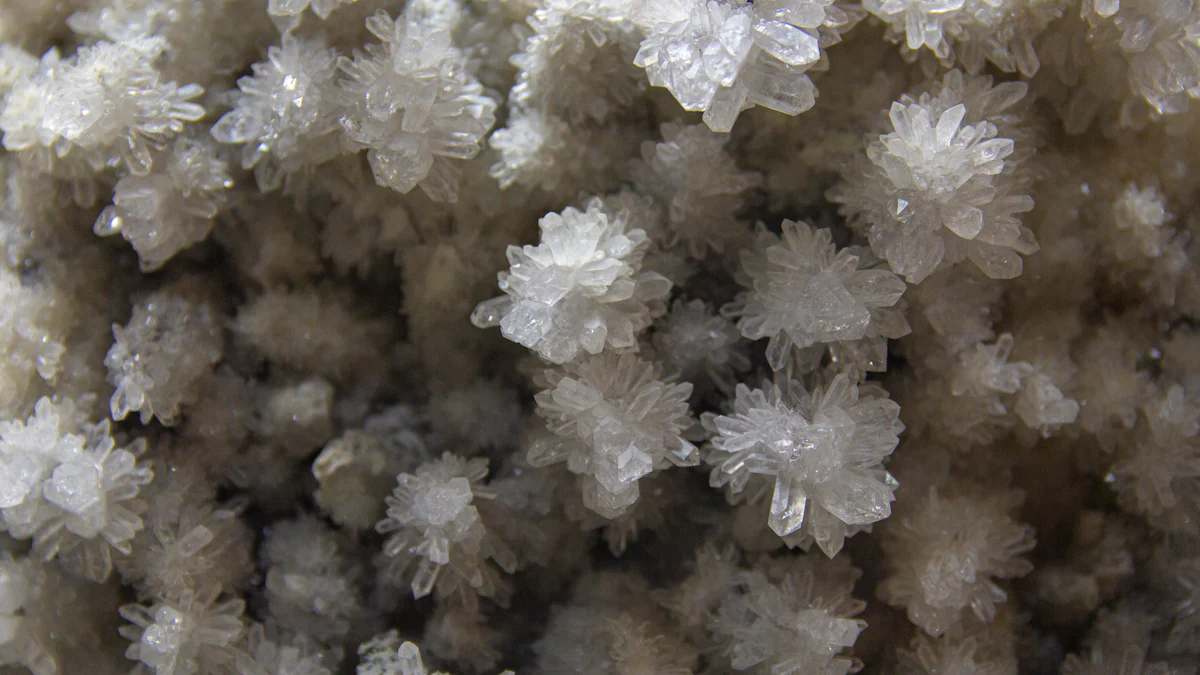
Epitaxy comes in various forms, each tailored to specific applications and material requirements. Understanding these types helps in appreciating their unique roles in advancing technology.
Homoepitaxy
Definition and Characteristics
Homoepitaxy refers to the growth of a crystalline film on a substrate made of the same material. This process ensures a seamless match between the film and the substrate, resulting in a uniform and defect-free layer. For example, growing silicon on a silicon substrate is a common practice in this method. The compatibility between the materials minimizes structural mismatches, making homoepitaxy ideal for applications requiring high purity and precision.
This type of epitaxy focuses on maintaining consistency in material properties. Engineers often rely on it to create layers with enhanced electrical conductivity or improved surface quality. Its ability to produce flawless crystalline structures makes it indispensable in industries that demand perfection.
Common Applications in Semiconductors
Homoepitaxy plays a vital role in semiconductor manufacturing. It is widely used to create high-quality silicon wafers, which serve as the foundation for many electronic devices. These wafers are essential for producing transistors, diodes, and integrated circuits. The uniformity achieved through homoepitaxy ensures that these components perform reliably under various conditions.
Additionally, homoepitaxy supports the development of advanced semiconductor devices. By providing a defect-free base, it enhances the efficiency and longevity of these devices. This method remains a cornerstone in the production of high-performance electronics.
Heteroepitaxy
Definition and Characteristics
Heteroepitaxy involves growing a crystalline layer on a substrate composed of a different material. Unlike homoepitaxy, this method introduces versatility by combining materials with distinct properties. For instance, gallium nitride (GaN) grown on a sapphire substrate exemplifies heteroepitaxy. This approach allows engineers to create materials that cannot be obtained naturally as single crystals.
The mismatch between the substrate and the film can pose challenges, but it also opens doors to innovation. Heteroepitaxy enables the design of materials with unique optical, electrical, or mechanical properties. This flexibility makes it a preferred choice for creating advanced materials.
Use in Creating Advanced Materials with Different Properties
Heteroepitaxy is crucial in developing optoelectronic devices and bandgap-engineered materials. It allows for the creation of structures like quantum wells and superlattices, which are essential for modern technologies. For example, LEDs and laser diodes rely on heteroepitaxial layers to achieve specific emission wavelengths.
This method also supports the production of materials with tailored properties. By carefully selecting the substrate and film combination, engineers can design devices that meet precise performance criteria. Heteroepitaxy continues to drive innovation in fields like photonics and renewable energy.
Molecular Beam Epitaxy (MBE)
Overview of the Process
Molecular Beam Epitaxy (MBE) is a highly controlled technique for growing thin crystalline layers. In this process, molecular or atomic beams are directed onto a heated substrate in an ultra-high vacuum environment. The atoms or molecules condense on the substrate, forming a crystalline layer with exceptional precision.
MBE stands out for its ability to grow layers one atomic layer at a time. This level of control allows researchers to create materials with unparalleled accuracy. The process operates at relatively low temperatures, reducing the risk of damaging the substrate or introducing defects.
Advantages in Precision and Control
MBE offers several advantages, particularly in research and development. Its precision makes it ideal for creating complex structures like quantum dots and heterostructures. Scientists use MBE to study material properties and develop new technologies.
This technique also excels in producing materials for optoelectronic devices. For instance, MBE is instrumental in fabricating high-performance lasers and detectors. Its ability to control layer thickness and composition ensures optimal device performance.
Moreover, MBE supports the use of applied materials epitaxy trays, which enhance the uniformity and quality of the grown layers. These trays play a critical role in maintaining the precision required for advanced applications.
Chemical Vapor Deposition (CVD)
Overview of the Process
Chemical Vapor Deposition (CVD) is a widely used technique for growing thin crystalline layers. In this process, gaseous precursors react or decompose on a heated substrate, forming a solid film. The reaction occurs in a controlled environment, ensuring precise material deposition. CVD stands out for its ability to produce uniform layers with excellent adhesion to the substrate.
This method offers remarkable versatility. Engineers can adjust parameters like temperature, pressure, and gas composition to tailor the properties of the deposited film. CVD works well for creating materials with specific electrical, optical, or mechanical characteristics. Its adaptability makes it a go-to choice for various industries.
Applications in Large-Scale Production
CVD plays a crucial role in large-scale semiconductor manufacturing. It enables the production of high-quality silicon wafers, which serve as the foundation for electronic devices. These wafers are essential for transistors, diodes, and integrated circuits. The scalability of CVD ensures consistent results, even in mass production.
Optoelectronics also benefits from CVD. This technique helps create materials for LEDs, solar cells, and photodetectors. For instance, vapor-phase epitaxy, a form of CVD, is commonly used to deposit silicon epilayers. These layers enhance the performance and efficiency of optoelectronic devices.
CVD’s efficiency and precision make it indispensable in modern technology. Its ability to produce defect-free films supports the development of advanced materials, driving innovation in electronics and photonics.
Liquid-Phase Epitaxy
Description of the Process
Liquid-Phase Epitaxy (LPE) involves growing crystalline layers from a liquid solution. In this process, a substrate is dipped into a molten solution containing the desired material. As the solution cools, the material crystallizes on the substrate, forming a thin layer. This method allows for controlled growth, resulting in high-quality films.
LPE operates at relatively low temperatures, reducing the risk of thermal damage to the substrate. It is particularly effective for creating thick layers with minimal defects. Engineers often use LPE to grow materials that are difficult to produce using other techniques.
Applications in Optoelectronics and Photonics
LPE is a key player in optoelectronics and photonics. It is widely used to manufacture materials for LEDs, laser diodes, and optical waveguides. These devices rely on the precise optical properties of epitaxial layers to function effectively.
For example, LPE helps create gallium arsenide (GaAs) and indium phosphide (InP) layers, which are essential for high-performance optoelectronic devices. These materials enable efficient light emission and detection, making them ideal for communication and display technologies.
The simplicity and reliability of LPE make it a preferred choice for specific applications. Its ability to produce high-quality layers supports the advancement of photonic technologies, paving the way for innovations in lighting and telecommunications.
Solid-Phase Epitaxy
Description of the Process
Solid-Phase Epitaxy (SPE) involves transforming an amorphous or polycrystalline layer into a crystalline one. This process begins with depositing a non-crystalline film onto a substrate. The substrate is then heated, causing the atoms in the film to rearrange and align with the underlying crystal structure. SPE ensures a seamless transition from the amorphous state to a high-quality crystalline layer.
This method is highly controlled and minimizes defects. SPE is particularly useful for repairing damaged crystalline structures or improving the quality of existing layers. Its precision makes it a valuable tool in material science.
Use in Repairing Damaged Crystalline Structures
SPE excels in restoring damaged crystalline structures. It is often used in semiconductor manufacturing to repair defects caused by ion implantation. By recrystallizing the damaged layer, SPE enhances the material’s electrical and optical properties.
This technique also supports the development of advanced devices. For instance, SPE helps create high-performance transistors and memory chips. Its ability to refine material quality ensures reliable device operation.
SPE’s role in repairing and improving materials highlights its importance in modern technology. It provides a cost-effective solution for enhancing the performance of semiconductors and other crystalline materials.
Applications of Epitaxy
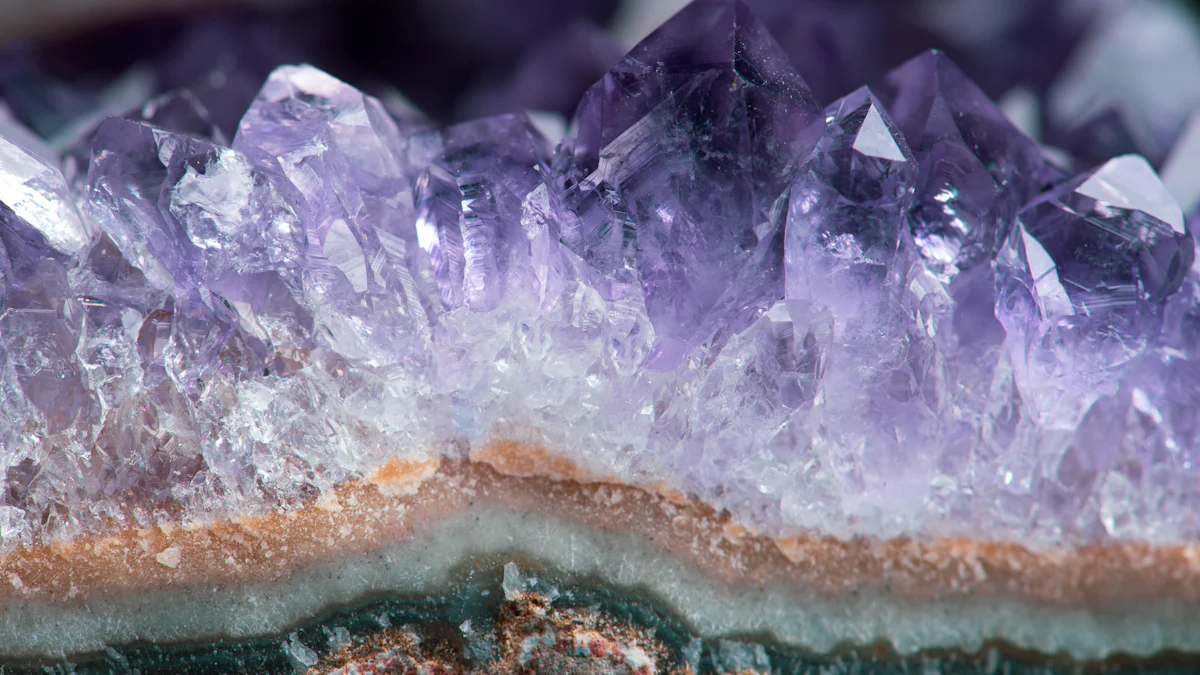
Epitaxy has revolutionized modern technology by enabling the creation of high-quality materials with precise properties. Its applications span across industries, driving advancements in semiconductors, optoelectronics, and emerging technologies.
Role in Semiconductor Manufacturing
Creation of High-Performance Chips
Epitaxy plays a critical role in producing high-performance semiconductor chips. By providing a flawless crystalline foundation, it ensures that materials transmit electrons efficiently. This precision enhances the speed and reliability of chips used in devices like smartphones, computers, and automotive systems. The demand for advanced semiconductor devices, fueled by innovations in 5G, artificial intelligence, and IoT, continues to grow. Epitaxy supports this demand by enabling scalable production methods that maintain quality even in mass manufacturing.
Use in Transistors and Integrated Circuits
Transistors and integrated circuits rely on epitaxial layers for optimal performance. These layers improve electrical conductivity and reduce defects, ensuring the devices operate consistently under various conditions. Epitaxy allows engineers to design multi-level and multifunctional devices, which are essential for compact and efficient electronics. The ability to control material properties precisely makes epitaxy indispensable in semiconductor fabrication.
Use in Optoelectronics
Development of LEDs and Laser Diodes
Epitaxy has transformed optoelectronics by enabling the development of LEDs and laser diodes. These devices depend on epitaxial layers to achieve specific optical properties, such as emitting light at precise wavelengths. For example, gallium nitride layers grown through epitaxy are crucial for creating bright and energy-efficient LEDs. Laser diodes, used in communication and medical devices, also benefit from the precision and quality of epitaxial materials.
Applications in Solar Cells
Solar cell technology relies heavily on epitaxy to enhance efficiency. Epitaxial layers improve the ability of solar cells to capture and convert sunlight into electricity. Materials like silicon and gallium arsenide, grown using epitaxy, enable the production of high-performance photovoltaic cells. These advancements contribute to the growth of renewable energy solutions, making solar power more accessible and sustainable.
Advanced Materials and Emerging Technologies
Use in Quantum Computing
Quantum computing represents the frontier of technological innovation, and epitaxy plays a vital role in its development. Epitaxial layers are used to create quantum dots and other nanostructures essential for quantum processors. These materials exhibit unique properties that enable faster and more secure data processing. By supporting the precise fabrication of quantum devices, epitaxy drives progress in this groundbreaking field.
Role in Nanotechnology and 2D Materials
Nanotechnology and 2D materials have unlocked new possibilities in material science, and epitaxy is at the heart of these advancements. It enables the growth of ultra-thin layers with exceptional precision, paving the way for innovations in electronics, sensors, and energy storage. For instance, epitaxy facilitates the production of graphene and other 2D materials, which are known for their remarkable strength and conductivity. These materials are shaping the future of technology, from flexible displays to advanced batteries.
Epitaxy’s versatility and precision make it a cornerstone of modern innovation. Whether in semiconductors, optoelectronics, or emerging fields like quantum computing, its impact continues to grow. Tools like applied materials epitaxy trays further enhance the quality and uniformity of epitaxial layers, ensuring consistent results across applications.
Advantages and Challenges of Epitaxy
Advantages
Precision in Material Growth
Epitaxy offers unmatched precision in growing crystalline layers. This process ensures that the atomic structure of the new layer aligns perfectly with the substrate beneath it. By achieving this level of accuracy, epitaxy minimizes structural defects and enhances material quality. For instance, in semiconductor manufacturing, this precision allows materials to transmit electrons more efficiently, which directly improves the performance of devices like microchips and transistors.
“Epitaxy enables the creation of defect-free, high-quality crystalline layers, which are essential for advanced electronic and optoelectronic devices.”
This ability to grow flawless layers makes epitaxy indispensable in industries that demand high-performance materials. Whether it’s for LEDs, solar cells, or integrated circuits, the precision of epitaxy ensures reliability and efficiency.
Ability to Create Custom Material Properties
Epitaxy provides engineers with the unique ability to design materials with tailored properties. By carefully controlling the growth process, they can adjust electrical, optical, or mechanical characteristics to meet specific requirements. For example, epitaxy allows the creation of materials that emit light at precise wavelengths, which is crucial for LEDs and laser diodes.
This customization extends to advanced technologies like quantum computing and nanotechnology. Engineers can use epitaxy to develop quantum dots or 2D materials with unique properties that drive innovation in these fields. The flexibility to create custom materials opens doors to new possibilities in technology and science.
Challenges
High Cost and Complexity
Despite its advantages, epitaxy comes with significant challenges. The process requires highly specialized equipment and controlled environments, which drive up costs. For instance, techniques like Molecular Beam Epitaxy (MBE) operate in ultra-high vacuum conditions, making them expensive to implement and maintain. Additionally, the complexity of the process demands skilled professionals to ensure optimal results.
These factors make epitaxy less accessible for smaller-scale operations or emerging industries. While the benefits often outweigh the costs for large-scale manufacturers, the financial barrier remains a challenge for broader adoption.
Limitations in Scalability for Certain Methods
Scalability poses another hurdle for epitaxy, especially for methods like MBE that prioritize precision over speed. While these techniques excel in research and development, they struggle to meet the demands of mass production. For example, producing large quantities of high-quality materials for consumer electronics can be time-consuming and resource-intensive.
“Balancing precision and scalability remains a key challenge in epitaxy, particularly for industries aiming to meet global demand.”
Chemical Vapor Deposition (CVD) offers better scalability but may compromise on the level of control compared to MBE. This trade-off highlights the ongoing challenge of adapting epitaxy techniques to suit both research and industrial needs. Overcoming these limitations will be crucial for expanding the applications of epitaxy in the future.
Epitaxy stands as a cornerstone of modern material science, offering diverse methods like homoepitaxy, heteroepitaxy, and advanced techniques such as Molecular Beam Epitaxy and Chemical Vapor Deposition. Each type brings unique strengths, from creating defect-free layers for microchips to enabling precise optical properties in LEDs. This precision has revolutionized semiconductors and optoelectronics, driving innovations in consumer electronics, telecommunications, and renewable energy.
Looking ahead, epitaxy holds immense potential in emerging fields. Quantum computing and nanotechnology rely on its ability to craft materials with unparalleled accuracy. Tools like applied materials epitaxy trays will continue to enhance the quality and scalability of these advancements, shaping the future of technology.
FAQ
What is epitaxy?
Epitaxy refers to the process of growing a crystalline layer on a substrate where the crystal structure aligns perfectly with the underlying material. This alignment ensures the formation of high-quality layers with minimal defects. In semiconductor fabrication, epitaxy enables the creation of thin films with precise orientations. These films are essential for electronic and photonic devices. By controlling growth conditions, scientists can engineer materials with specific electrical, optical, or mechanical properties. This precision makes epitaxy a cornerstone of material science.
Why is epitaxy important in semiconductor manufacturing?
Epitaxy plays a critical role in semiconductor manufacturing. It precisely controls material properties, improves crystal quality, and enhances device performance. This process allows engineers to design multi-level and multifunctional devices, such as transistors and integrated circuits. Epitaxy also supports heterogeneous integration, enabling the combination of different materials to create advanced devices. Without epitaxy, modern electronic devices would lack the high performance and efficiency they require.
What are the main types of epitaxy?
Epitaxy can be categorized into several types, including homoepitaxy and heteroepitaxy. Homoepitaxy involves growing a crystalline layer on a substrate of the same material, such as silicon on silicon. This method ensures a seamless match and minimal defects. Heteroepitaxy, on the other hand, involves growing a layer on a substrate made of a different material, like gallium nitride on sapphire. This approach allows for the creation of materials with unique properties, making it ideal for advanced applications like LEDs and laser diodes.
How does Molecular Beam Epitaxy (MBE) differ from Chemical Vapor Deposition (CVD)?
Molecular Beam Epitaxy (MBE) and Chemical Vapor Deposition (CVD) are two popular epitaxy techniques. MBE uses molecular or atomic beams in an ultra-high vacuum environment to grow thin crystalline layers with exceptional precision. It is ideal for research and development due to its ability to control growth at the atomic level. CVD, on the other hand, involves chemical reactions of gaseous precursors on a heated substrate to form a solid film. CVD is widely used for large-scale production because of its efficiency and scalability.
What industries benefit from epitaxy?
Epitaxy benefits a wide range of industries, including semiconductors, optoelectronics, and renewable energy. In semiconductors, it supports the production of high-performance chips and transistors. Optoelectronics relies on epitaxy for devices like LEDs, laser diodes, and solar cells. Emerging fields such as quantum computing and nanotechnology also use epitaxy to create advanced materials like quantum dots and 2D materials. Its versatility makes it indispensable across multiple sectors.
Can epitaxy improve the efficiency of solar cells?
Yes, epitaxy significantly improves the efficiency of solar cells. By creating high-quality crystalline layers, epitaxy enhances the ability of solar cells to capture and convert sunlight into electricity. Materials like silicon and gallium arsenide, grown using epitaxy, enable the production of high-performance photovoltaic cells. These advancements contribute to the development of more efficient and sustainable renewable energy solutions.
What challenges does epitaxy face?
Epitaxy faces challenges such as high costs and complexity. The process requires specialized equipment and controlled environments, which increase expenses. Techniques like Molecular Beam Epitaxy (MBE) demand ultra-high vacuum conditions, making them resource-intensive. Scalability is another hurdle, especially for methods that prioritize precision over speed. Balancing these factors remains a key challenge for industries aiming to meet global demand while maintaining quality.
How does epitaxy enable the creation of custom materials?
Epitaxy allows engineers to design materials with tailored properties by controlling the growth process. They can adjust electrical, optical, or mechanical characteristics to meet specific requirements. For example, epitaxy enables the creation of materials that emit light at precise wavelengths, which is crucial for LEDs and laser diodes. This customization supports innovations in advanced technologies like quantum computing and nanotechnology.
What is the difference between homoepitaxy and heteroepitaxy?
Homoepitaxy involves growing a crystalline layer on a substrate of the same material, ensuring a seamless match and minimal defects. For instance, silicon grown on silicon is a common example. Heteroepitaxy, however, involves growing a layer on a substrate made of a different material. This method introduces versatility by combining materials with distinct properties, such as gallium nitride on sapphire. Heteroepitaxy enables the creation of advanced materials with unique optical and electrical characteristics.
How does epitaxy contribute to quantum computing?
Epitaxy plays a vital role in quantum computing by enabling the creation of quantum dots and other nanostructures. These materials exhibit unique properties that support faster and more secure data processing. Epitaxial layers provide the precision needed to fabricate quantum devices, driving progress in this groundbreaking field. By supporting the development of quantum processors, epitaxy helps shape the future of computing.