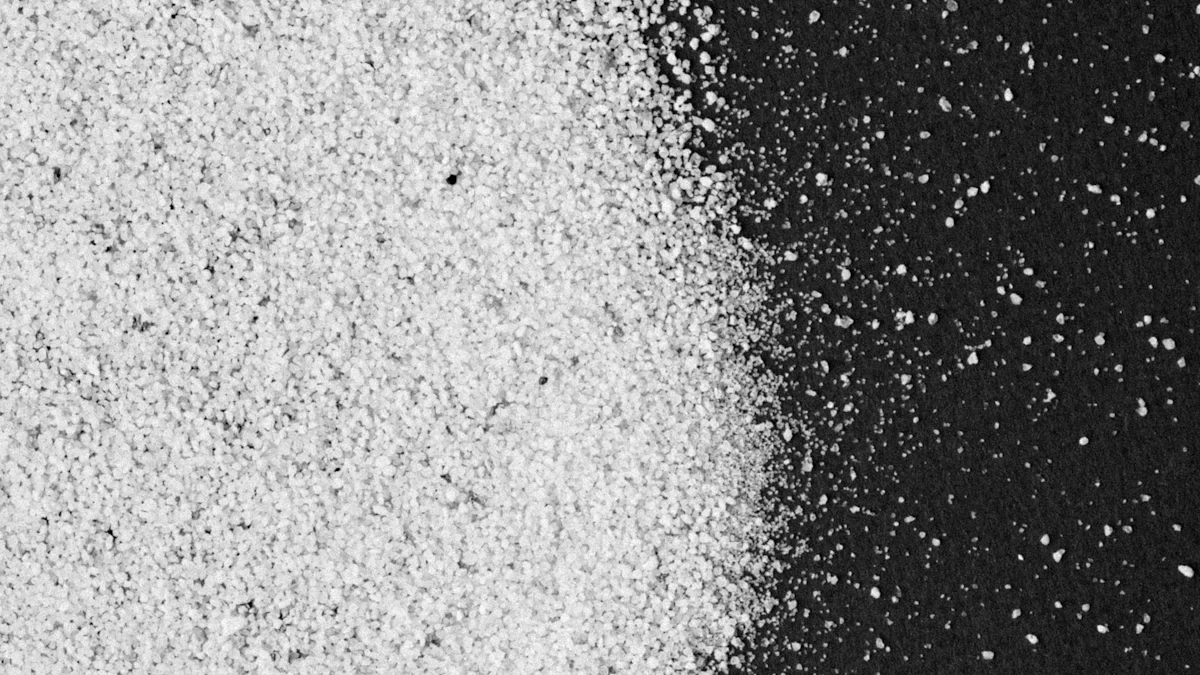
Silicon carbide continues to revolutionize industries, but its journey isn’t without hurdles. In 2025, its adoption faces roadblocks like processing difficulties, electrical resistance issues, and thermal shock limitations. Despite these challenges, the global silicon carbide market is booming. It’s projected to grow from 3.68billionin2023to3.68 billion in 2023 to 3.68billionin2023to7.98 billion by 2030, driven by demand in automotive and aerospace sectors. Applications like Silicon Carbide Bearings in jet turbines and Silicon Carbide Granules for abrasives highlight its versatility. However, these advancements depend on overcoming its inherent material challenges.
Key Takeaways
- Silicon carbide is very hard and brittle, needing special tools to shape.
- Making it costs a lot because materials and energy are pricey.
- Problems like tiny cracks and flaws can hurt how devices work.
- It doesn’t handle heat well, which lowers its power efficiency.
- Quick temperature changes can break it due to thermal shock.
- Getting materials depends on certain areas, which can cause delays.
- Making silicon carbide creates a lot of pollution, needing cleaner ways.
- Better methods and teamwork are key to fixing these problems.
Processing Challenges of Silicon Carbide
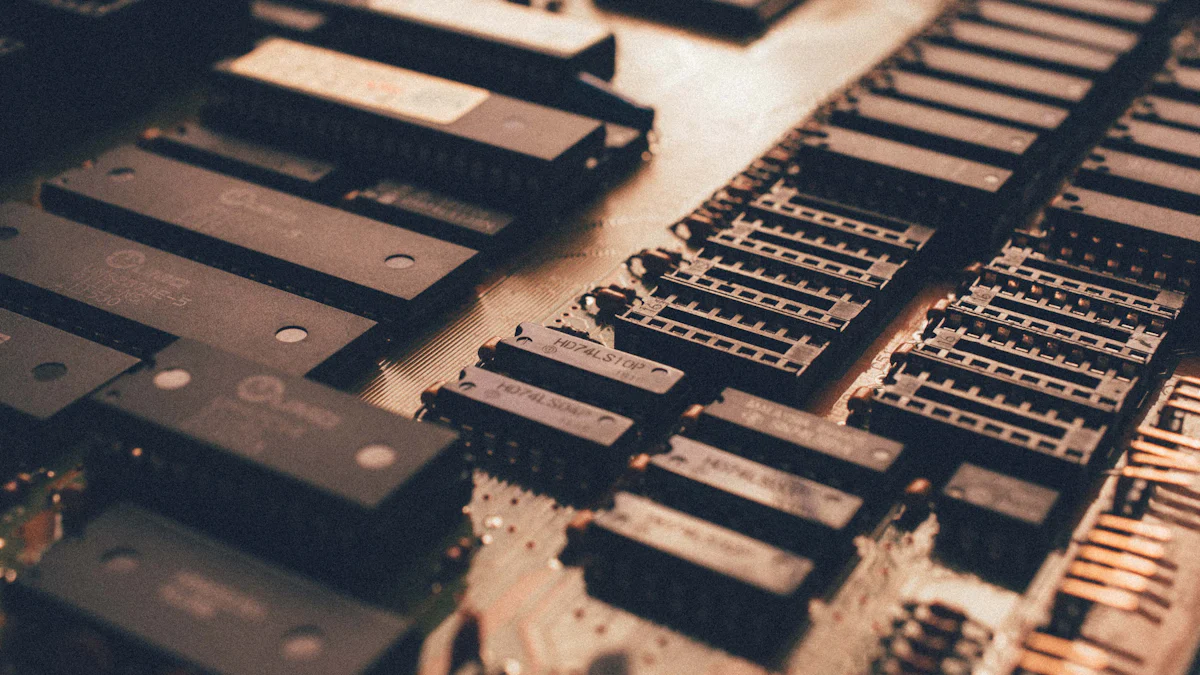
Hardness and Brittleness
Impact on machining and shaping processes
Silicon carbide is incredibly hard, which makes it both a blessing and a challenge. Its hardness requires specialized diamond grinding techniques for machining. However, its brittleness complicates the process further. During machining, the material is prone to sub-surface damage and micro-cracks. These imperfections can weaken the final product, leading to premature failure when subjected to operational stresses. Improper machining methods only amplify these risks, making precision an absolute necessity.
Need for specialized tools and techniques
Working with silicon carbide demands more than just standard tools. Manufacturers rely on advanced equipment like diamond-coated tools to shape and machine the material effectively. While these tools get the job done, they come with a hefty price tag. The need for such specialized techniques increases production costs and limits accessibility for smaller manufacturers.
High Manufacturing Costs
Expensive raw materials and production processes
The production of silicon carbide involves costly raw materials like petroleum coke and silicon metal. Prices for these materials fluctuate based on supply and demand, adding unpredictability to manufacturing expenses. The process itself is energy-intensive, requiring high-temperature sintering that consumes significant resources. Additionally, achieving high-purity silicon carbide, essential for many applications, involves stringent manufacturing standards, which further drive up costs.
Limited scalability for mass production
High manufacturing costs make it difficult to scale silicon carbide production for mass markets. The complexity of the process limits economies of scale, keeping costs per unit high. For instance, the energy-intensive sublimation process and yield issues—such as unusable wafers—add to the overall expense. While larger production volumes could reduce costs, achieving this scale remains a challenge due to the premium pricing of high-purity materials and the intricate manufacturing steps involved.
Common Defects in Silicon Carbide Substrates
Crystalline stacking faults and micropipes
Silicon carbide substrates often suffer from defects like basal plane dislocations, micropipes, and stacking faults. These imperfections directly impact the performance of electronic devices. For example, micropipes can limit the operational current and increase leakage, while stacking faults reduce blocking voltage and compromise reliability. Such defects make it challenging to produce substrates that meet the high standards required for advanced applications.
Challenges in achieving defect-free substrates
Eliminating defects in silicon carbide substrates is no easy feat. Advanced growth technologies, such as chemical vapor deposition, have made strides in improving crystal quality. However, achieving completely defect-free substrates remains a work in progress. The presence of surface scratches, polytype inclusions, and other imperfections continues to pose hurdles for manufacturers aiming to deliver reliable, high-performance components.
Electrical Resistance Issues in Silicon Carbide
Performance at High Temperatures
Degradation of electrical properties under extreme heat
Silicon carbide stands out for its ability to handle high temperatures, but it’s not entirely immune to challenges. When exposed to extreme heat, its electrical properties can degrade over time. This degradation impacts the material’s efficiency, especially in applications like power electronics. Devices relying on silicon carbide may experience reduced performance if the material’s electrical stability weakens under prolonged heat exposure.
Impact on efficiency in power electronics
Despite these challenges, silicon carbide performs better than traditional silicon in high-temperature environments. Its thermal stability allows it to maintain electrical performance where silicon would fail. This makes it a preferred choice for high-power and high-frequency applications, such as electric vehicle inverters and industrial power systems. However, manufacturers must address the gradual loss of efficiency to ensure long-term reliability in these demanding applications.
Threshold Voltage Failures
Prolonged gate signal application in power MOSFETs
Power MOSFETs made from silicon carbide face unique reliability issues. One major concern is threshold voltage failure, which occurs when the gate signal is applied for extended periods. This can lead to gate oxide breakdown due to the thinner oxide layer and higher electric fields in silicon carbide devices. Over time, this breakdown increases the threshold voltage, making the MOSFET less reliable.
Reliability concerns in long-term applications
Threshold voltage failures don’t just affect short-term performance—they raise concerns about long-term reliability. Devices that rely on silicon carbide MOSFETs may experience inconsistent operation as the threshold voltage shifts. However, regular switching of the gate voltage between on and off states can help reverse these changes, improving the device’s lifespan. Engineers are exploring ways to mitigate this issue further, ensuring silicon carbide remains a dependable option for power electronics.
Environmental Sensitivity
Susceptibility to oxidation and chemical reactions
Silicon carbide’s performance can also be affected by its sensitivity to environmental factors. It’s prone to oxidation and chemical reactions, especially in harsh conditions. These reactions can alter the material’s surface properties, leading to reduced efficiency or even failure in some cases.
Need for protective coatings or treatments
To combat this, manufacturers often apply protective coatings or treatments to silicon carbide components. These coatings shield the material from oxidation and other environmental damage, extending its lifespan. While effective, these additional steps increase production costs and complexity. Researchers are working on more cost-effective solutions to enhance silicon carbide’s durability without compromising its performance.
Thermal Shock Resistance Limitations of Silicon Carbide
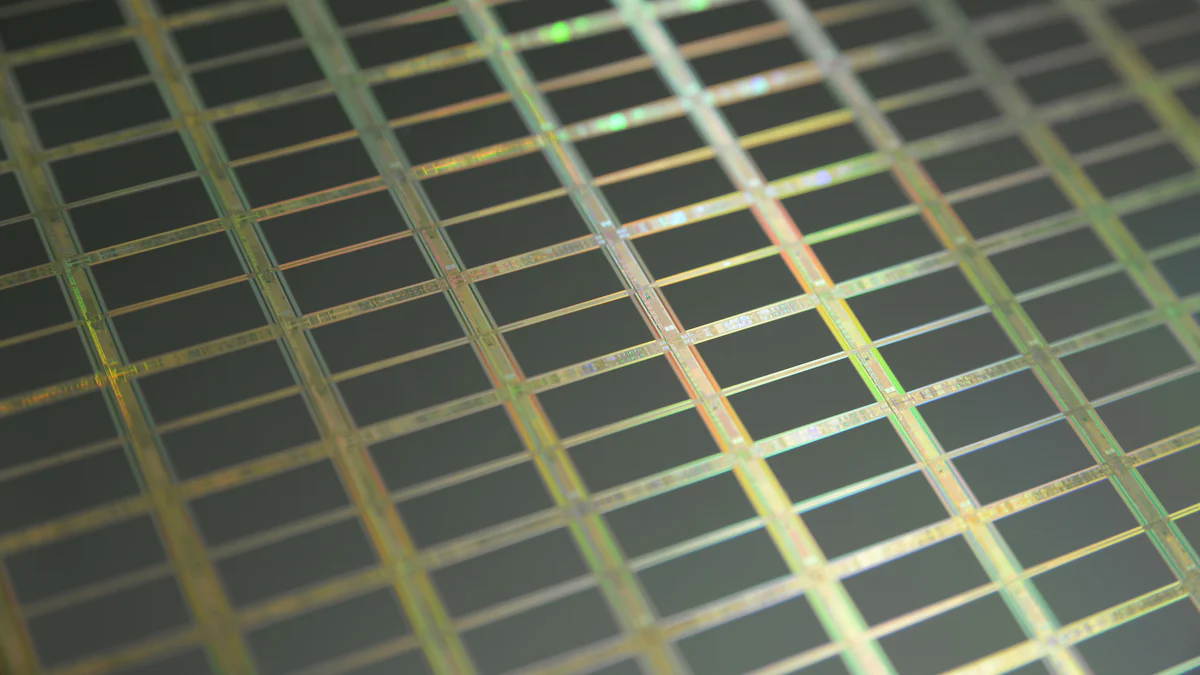
Vulnerability to Rapid Temperature Changes
Risks of cracking and structural damage
Silicon carbide is known for its strength and durability, but it struggles with rapid temperature changes. When exposed to sudden shifts in temperature, the material can develop thermal stresses. These stresses often lead to microcracks or even larger fractures. Over time, these cracks weaken the structure, making it more prone to failure. This vulnerability limits its use in applications where temperature fluctuations are frequent or extreme.
Impact on long-term durability
Thermal shock doesn’t just cause immediate damage; it also affects the material’s lifespan. Repeated exposure to rapid heating and cooling cycles can gradually degrade silicon carbide’s structural integrity. This makes it less reliable for long-term use, especially in industries like aerospace or energy, where durability is critical. Manufacturers must address this issue to ensure the material performs consistently over time.
Challenges in High-Temperature Applications
Limitations in thermal management systems
Silicon carbide performs well at high temperatures, but managing heat effectively remains a challenge. In many systems, the material’s thermal conductivity helps dissipate heat. However, when combined with its sensitivity to thermal shock, this advantage becomes less effective. Designing thermal management systems that balance heat dissipation with structural protection is a complex task. Engineers often need to incorporate additional materials or cooling mechanisms, which increases costs and design complexity.
Reduced performance in energy-intensive industries
Industries like power generation and manufacturing rely on materials that can handle extreme conditions. While silicon carbide offers excellent thermal stability, its limitations in thermal shock resistance can reduce its performance in these demanding environments. For example, in furnaces or reactors, rapid temperature changes can compromise the material’s efficiency and reliability. This makes it less suitable for some energy-intensive applications, despite its other advantages.
Comparison with Alternative Materials
Advantages and disadvantages of silicon carbide vs. other materials
Silicon carbide stands out for its high thermal conductivity and ability to withstand elevated temperatures. However, it falls short compared to some alternative materials when it comes to thermal shock resistance. Quick temperature changes can create thermal stresses, leading to microcracks that compromise the material’s integrity.
Potential trade-offs in material selection
When choosing materials for high-temperature applications, engineers must weigh the pros and cons. Here’s a quick comparison:
Advantages of SiC | Limitations of SiC |
---|---|
Exceptional thermal stability and resistance to high temperatures | Higher cost compared to silicon |
Higher breakdown voltages and lower on-resistance | Complex processing requirements |
Robust performance in extreme environments | Reliability concerns in long-term applications |
Silicon carbide excels in high-pressure and high-temperature environments. Its wide-bandwidth characteristics and high carrier mobility make it ideal for high-frequency and high-power applications. It also enhances energy efficiency in electric vehicles and renewable energy systems. However, its higher cost and processing challenges often lead manufacturers to consider alternatives.
Supply Chain and Market Dynamics of Silicon Carbide
Limited Raw Material Sources
Dependence on specific regions for production
The production of silicon carbide relies heavily on specific regions rich in raw materials like silicon and carbon. Countries with advanced manufacturing capabilities dominate the supply chain, creating a geographical imbalance. This dependence makes the industry vulnerable to regional disruptions, such as political instability or trade restrictions. For companies relying on silicon carbide, this uneven distribution poses a significant challenge in maintaining a steady supply.
Risks of supply chain disruptions
The global supply chain for semiconductors, including silicon carbide, faces risks from various factors. Geopolitical tensions, natural disasters, and resource shortages can all disrupt the flow of critical materials. These risks are particularly concerning for silicon carbide due to its reliance on specialized resources. To address these vulnerabilities, companies are forming strategic partnerships and diversifying their supply chains. Such efforts aim to reduce the impact of potential disruptions and ensure consistent production.
Manufacturing Bottlenecks
Challenges in scaling up production
Scaling up silicon carbide production is no easy task. The manufacturing process involves energy-intensive steps and requires advanced equipment, which limits the ability to increase output quickly. High-purity silicon carbide, essential for many applications, demands stringent quality control, further complicating the process. These challenges slow down production and make it difficult to meet the growing demand in industries like automotive and electronics.
Impact on global demand and pricing
Manufacturing bottlenecks have a direct impact on the global market.
- Supply shortages often lead to higher prices, making silicon carbide less accessible for smaller manufacturers.
- The energy-intensive nature of production adds to these bottlenecks, increasing costs further.
- As demand for silicon carbide rises in sectors like power electronics and renewable energy, these bottlenecks create a ripple effect, influencing both availability and pricing worldwide.
Competition with Alternative Materials
Growing interest in other semiconductors
Silicon carbide faces stiff competition from alternative materials like gallium nitride (GaN). These materials offer unique advantages, such as lower production costs and fewer defects during manufacturing. While silicon carbide excels in high-temperature and high-power applications, its brittleness and complex production process make it less appealing in some cases. This growing interest in alternatives challenges silicon carbide’s dominance in the semiconductor market.
Market dynamics and adoption trends
The competition between silicon carbide and other materials is shaping market trends. Factors like defect rates, production costs, and material properties play a significant role in determining adoption rates. For instance, gallium nitride’s lower defect rates and simpler manufacturing process make it an attractive option for many applications. However, silicon carbide remains a strong contender due to its superior performance in extreme conditions. As industries continue to innovate, the balance between these materials will likely shift, influencing future market dynamics.
Environmental and Sustainability Concerns with Silicon Carbide
Energy-Intensive Production Processes
High carbon footprint of manufacturing
Producing silicon carbide requires a lot of energy, which leaves a significant carbon footprint. Processes like Polymer Infiltration Pyrolysis (PIP) have the highest environmental impact, while Chemical Vapor Infiltration (CVI) is less harmful. However, all methods contribute to greenhouse gas emissions, especially carbon dioxide. Mining for raw materials like tungsten and cobalt adds to the problem. It depletes resources, disrupts habitats, and causes pollution. These factors make the production process a major environmental concern.
Need for greener production methods
To address these issues, researchers are exploring greener production methods. A new process has shown promise by cutting CO2 emissions by 75%. It also eliminates toxic byproducts like sulfur oxides and nitrogen oxides. This method uses natural gas and industrial silicon waste to create high-purity silicon carbide powder. As a bonus, it generates hydrogen, a clean energy source. With production costs estimated at 10to10 to 10to20 per kilogram, this approach could make manufacturing more sustainable and affordable.
Recycling and End-of-Life Challenges
Difficulty in recycling silicon carbide components
Recycling silicon carbide components is no easy task. The material’s durability, while beneficial during use, makes it hard to break down and repurpose. This creates challenges for industries looking to recycle and reuse silicon carbide products. Without effective recycling methods, old components often end up in landfills, contributing to waste.
Environmental impact of disposal
Disposing of silicon carbide components has environmental consequences. On the bright side, longer-lasting products like silicon carbide crucibles reduce waste. They don’t need frequent replacements, which lowers landfill contributions. Their high thermal conductivity also improves energy efficiency during use, reducing the carbon footprint. Extending the lifespan of these components conserves resources and minimizes the impact of raw material extraction. However, finding ways to recycle them remains crucial for long-term sustainability.
Regulatory and Compliance Issues
Stricter environmental regulations in 2025
In 2025, stricter environmental regulations are expected to reshape the silicon carbide industry. Governments are pushing for greener practices, urging manufacturers to innovate. These changes align with growing consumer demand for eco-friendly products. Companies must adapt to meet these standards while maintaining production efficiency.
Impact on production and adoption
These regulations could increase production costs but also drive innovation. The rising need for power electronics in electric vehicles and renewable energy sectors highlights the importance of silicon carbide. By adopting sustainable practices, manufacturers can ensure the material’s continued use in these critical industries. Balancing compliance with affordability will be key to its broader adoption.
Potential Solutions and Future Outlook for Silicon Carbide
Advances in Manufacturing Techniques
Development of cost-effective and scalable processes
Reducing the cost and improving the scalability of silicon carbide production requires innovative approaches. Several advancements are already making a difference:
- Efficient growth technologies, like sublimation and chemical vapor deposition, improve crystal quality and reduce defects. This lowers production costs and enhances the reliability of silicon carbide components.
- Thin-sheet technology allows for precision cutting, creating thinner sheets that reduce material waste and improve device performance. This innovation supports scalability by optimizing resource use.
- New doping techniques enhance the efficiency of silicon carbide devices. These processes optimize electrical performance, making the material more cost-effective for mass production.
- Automation and intelligent manufacturing systems, such as real-time monitoring and AI-driven optimization, streamline production. These technologies boost efficiency and enable larger production volumes, meeting the growing demand across industries.
Innovations in machining and shaping technologies
Machining silicon carbide remains a challenge due to its hardness and brittleness. To address this, manufacturers rely on diamond grinding techniques, which are effective but require skilled operators. Proper handling is crucial to avoid sub-surface damage or micro-cracks that could weaken the material. Additionally, the sintering process benefits from the use of sintering aids, which help bond silicon carbide grains more effectively. These innovations ensure the material maintains its structural integrity while reducing the risk of defects during machining.
Material Enhancements
Research into improving thermal and electrical properties
Researchers are exploring ways to enhance silicon carbide’s thermal and electrical performance. By refining its crystal structure, they aim to reduce defects like micropipes and stacking faults. These improvements could significantly boost the material’s efficiency in high-power and high-temperature applications. Additionally, advancements in doping techniques are helping to optimize electrical conductivity, making silicon carbide even more reliable for demanding industries like automotive and aerospace.
Development of hybrid materials or composites
Combining silicon carbide with other materials offers exciting possibilities. Hybrid materials or composites can address some of its limitations, such as brittleness and thermal shock resistance. For example, integrating silicon carbide with metals or polymers could create components that are both strong and flexible. These composites would expand the range of applications for silicon carbide, making it a more versatile choice for engineers.
Industry Collaboration and Investment
Partnerships to address supply chain challenges
Collaboration across the industry is essential to overcome supply chain issues. Initiatives like the CHIPS Act focus on onshoring semiconductor production, reducing reliance on foreign suppliers. Companies like Onsemi are also expanding their production capabilities by acquiring dedicated silicon carbide manufacturing facilities. Strategic partnerships with raw material suppliers and technology providers ensure a steady supply of high-quality materials, minimizing the risk of disruptions.
Increased funding for research and development
Investment in research and development is driving innovation in silicon carbide technology. Governments and private companies are prioritizing funding to improve manufacturing processes and material properties. These efforts not only address current challenges but also position silicon carbide as a leader in next-generation technologies. By fostering collaboration and innovation, the industry can ensure a sustainable and competitive future for silicon carbide.
Silicon carbide offers remarkable benefits but comes with its share of challenges. Its processing complexity, electrical resistance issues, and thermal shock limitations hinder its broader adoption. Overcoming these obstacles demands innovation in manufacturing techniques, material improvements, and stronger industry partnerships. With ongoing advancements, silicon carbide could redefine industries like automotive and aerospace. Its potential to drive future technologies makes it a material worth investing in and improving.
FAQ
What makes silicon carbide so hard to process?
Silicon carbide’s extreme hardness and brittleness make it tough to machine. Manufacturers need diamond-coated tools and precise techniques to avoid cracks or damage. These specialized methods increase production costs and complexity.
Why is silicon carbide expensive to produce?
Its production involves costly raw materials like silicon metal and energy-intensive processes. High-purity silicon carbide requires advanced manufacturing techniques, which add to the expense. Scaling up production remains a challenge, keeping costs high.
How does silicon carbide compare to gallium nitride?
Silicon carbide excels in high-temperature and high-power applications. Gallium nitride, however, offers lower production costs and fewer defects. Engineers choose between them based on application needs, balancing performance and cost.
Can silicon carbide handle rapid temperature changes?
Not well. Rapid temperature shifts can cause thermal stress, leading to cracks or structural damage. This limits its use in environments with frequent or extreme temperature fluctuations.
Is silicon carbide environmentally friendly?
Its production has a high carbon footprint due to energy-intensive processes. Recycling silicon carbide is also difficult. However, researchers are developing greener methods to reduce emissions and improve sustainability.
What industries benefit most from silicon carbide?
Automotive, aerospace, and electronics industries rely heavily on silicon carbide. It’s used in electric vehicle inverters, jet turbines, and power electronics due to its high thermal stability and efficiency.
Are there solutions to silicon carbide’s defects?
Yes, advanced growth technologies like chemical vapor deposition help reduce defects like micropipes and stacking faults. Researchers are also exploring hybrid materials to improve its properties and reliability.
Will silicon carbide become more affordable?
With innovations in manufacturing and increased industry investment, costs could decrease over time. Automation and scalable processes may help make silicon carbide more accessible for mass production.
Tip: Keep an eye on industry advancements. Silicon carbide’s future depends on overcoming its current challenges through innovation and collaboration.