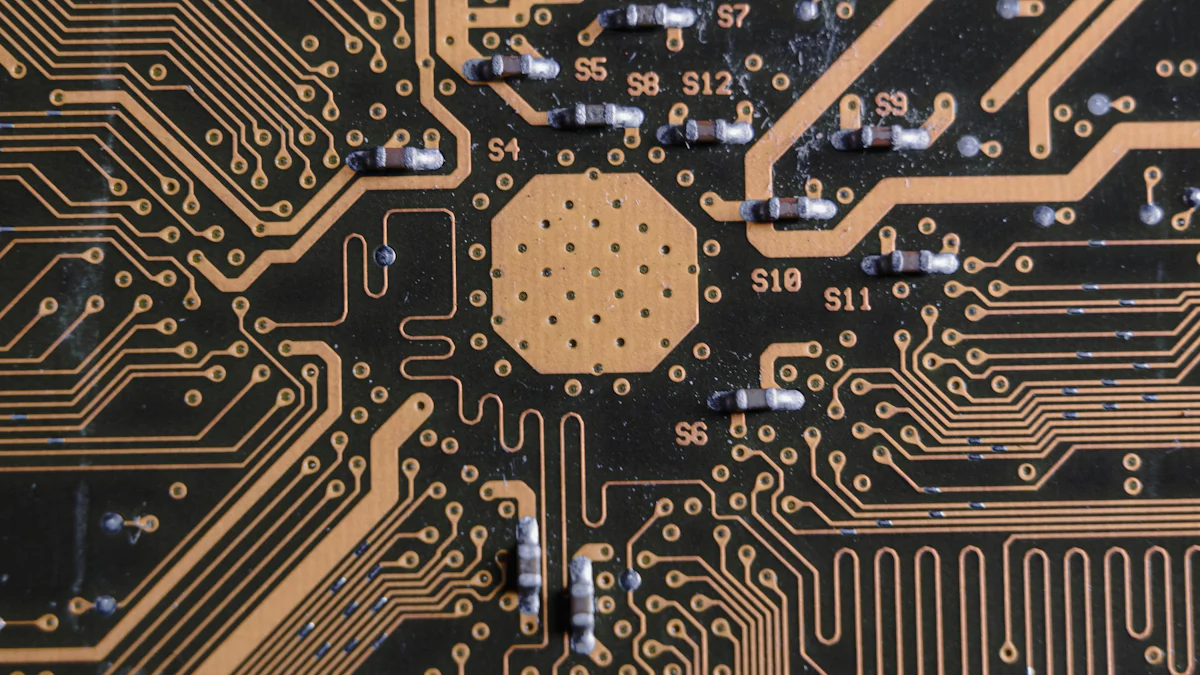
Epitaxial growth technology plays a pivotal role in shaping modern materials science. This process involves depositing a crystalline layer that aligns perfectly with the substrate beneath it, creating a seamless structure. Its precision enables the development of high-quality films with controlled properties, essential for advanced applications. In applied materials epitaxy, this technology enhances semiconductor performance, revolutionizing industries like electronics and photovoltaics. The use of applied materials epitaxy trays further optimizes the growth process, ensuring uniformity and efficiency. The demand for applied materials epi continues to rise, with the global market projected to reach $3,326.964 million by 2030. From semiconductors to nanotechnology, its transformative impact drives innovation across countless fields.
Key Takeaways
- Epitaxial growth technology is essential for creating high-quality crystalline materials, crucial for advancements in semiconductors and optoelectronics.
- Lattice matching ensures that the deposited layer aligns perfectly with the substrate, minimizing defects and enhancing material performance.
- Innovative methods like Van der Waals epitaxy and remote epitaxy allow for greater flexibility in material growth, enabling the use of 2D materials and substrate reusability.
- Molecular Beam Epitaxy (MBE) and Metal-Organic Chemical Vapor Deposition (MOCVD) are key techniques that provide precision and scalability for producing high-performance materials.
- The integration of AI in epitaxial growth processes can optimize conditions and accelerate material discovery, leading to more efficient production.
- Hybrid material systems combining traditional and 2D materials offer new opportunities for innovation in electronics, photonics, and energy applications.
- Addressing challenges like thermal misfit and defect reduction is vital for ensuring the stability and reliability of advanced materials.
Foundational Principles of Applied Materials Epitaxy
Understanding the foundational principles of applied materials epitaxy reveals how this technology achieves precision and efficiency in material growth. These principles ensure that the crystalline layers formed during the process exhibit exceptional quality and functionality. Let’s explore some of the key concepts that drive this innovative field.
Lattice Matching and Crystal Alignment
Lattice matching lies at the heart of epitaxial growth. It ensures that the crystal structure of the deposited layer aligns perfectly with the substrate beneath it. This alignment minimizes defects, which can otherwise compromise the material’s performance. For example, when growing semiconductor films, the lattice constants of the substrate and the film must closely match. This precise alignment enhances the electrical and mechanical properties of the final product.
Crystal alignment also plays a critical role in maintaining the structural integrity of the material. Misaligned crystals can lead to strain or thermal misfit, which may cause cracks or dislocations. By focusing on lattice matching, applied materials epitaxy achieves high-quality films suitable for advanced applications like semiconductors and optoelectronics.
Van der Waals Epitaxy in 2D Materials
Van der Waals epitaxy (vdWE) introduces a fascinating approach to material growth. Unlike traditional methods, vdWE allows crystalline films to grow on two-dimensional (2D) materials without requiring lattice matching. This flexibility opens up new possibilities for creating hybrid material systems. For instance, graphene, a well-known 2D material, serves as an excellent buffer layer for vdWE. Its unique properties enable the growth of high-quality films even when the substrate and film have mismatched lattice structures.
This method has gained significant attention in recent years due to its potential in next-generation devices. By leveraging vdWE, researchers can develop materials with tailored properties, paving the way for innovations in nanotechnology and quantum computing.
Remote Epitaxy and Substrate Reusability
Remote epitaxy takes the concept of material growth to another level. In this process, a thin 2D-material buffer layer separates the substrate from the growing film. Despite this separation, the substrate still influences the film’s growth through atomic interactions. This technique allows the film to maintain its structural relationship with the substrate while remaining physically detached.
One of the most exciting aspects of remote epitaxy is substrate reusability. After the film grows, it can be easily removed, leaving the substrate intact for future use. This approach not only reduces costs but also promotes sustainability in material production. Industries like semiconductors and photovoltaics benefit greatly from this innovation, as it enables scalable and efficient manufacturing processes.
“Remote epitaxy, in which the thin film is seeded by the underlying substrate through a 2D-material buffer layer, allows to unclamp epitaxial films from the substrate, while maintaining their structural relationship.” – Nature Electronics
By combining these principles, applied materials epitaxy continues to push the boundaries of what’s possible in material science. From lattice matching to remote epitaxy, each concept contributes to the creation of high-performance materials that drive technological advancements.
Advanced Methods in Epitaxial Growth Technology
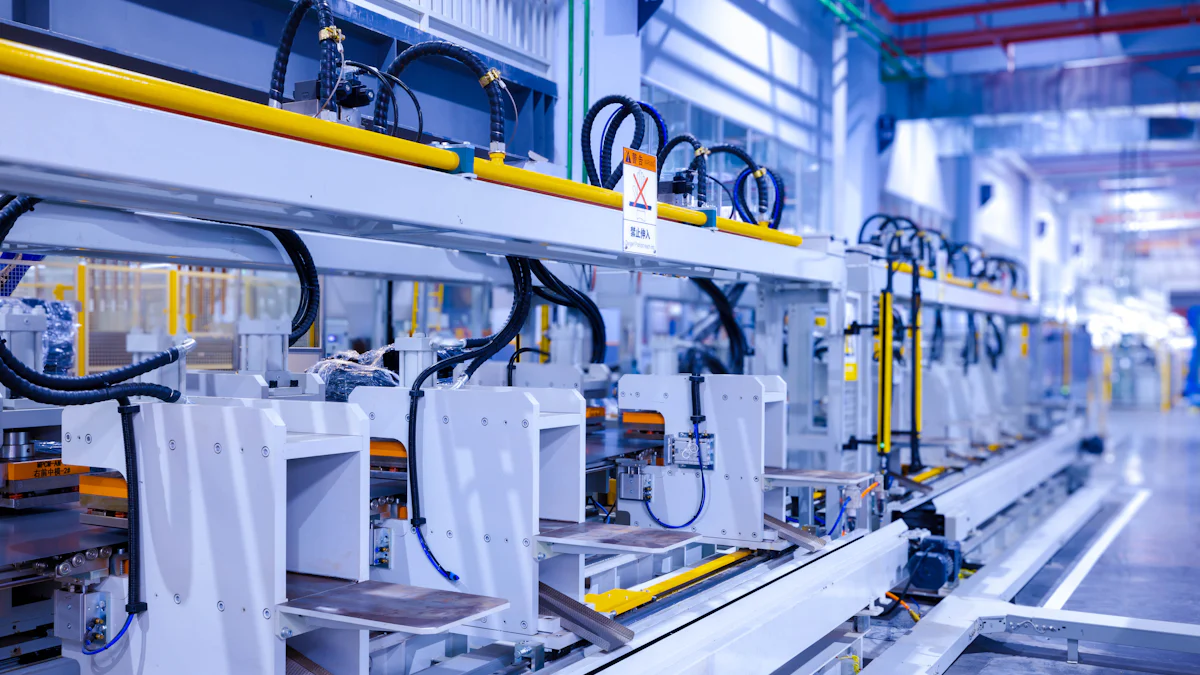
Epitaxial growth technology has evolved significantly, offering advanced methods that cater to diverse material needs. These methods ensure precision, efficiency, and adaptability, making them indispensable in applied materials epitaxy. Let’s dive into some of the most prominent techniques shaping this field.
Molecular Beam Epitaxy (MBE) for Precision Growth
Molecular Beam Epitaxy (MBE) stands out for its unparalleled precision. This method involves heating source materials in a high-vacuum environment to produce an evaporated beam of particles. These particles travel through the vacuum and deposit onto the substrate, forming a crystalline layer. The ultra-high vacuum ensures minimal contamination, allowing for atomic-level control over the growth process.
MBE is particularly valuable for research and development. Scientists use it to create highly crystalline films with precise control over thickness and composition. For example, compound semiconductors benefit greatly from this technique, as it enables the fabrication of intricate structures required for advanced electronic devices. Although MBE offers exceptional accuracy, its high cost often limits its use to specialized applications.
“MBE allows researchers to achieve atomic-level precision, making it a preferred choice for developing cutting-edge semiconductor materials.”
Pulsed Laser Deposition (PLD) for Complex Oxides
Pulsed Laser Deposition (PLD) provides a unique approach to growing complex oxide materials. In this method, a high-powered laser pulses onto a target material, vaporizing it into a plasma plume. This plume then deposits onto the substrate, forming a thin film. PLD excels in creating materials with intricate compositions, such as perovskites and spinels.
One of the key advantages of PLD is its versatility. It can handle a wide range of materials, making it ideal for producing films with tailored properties. Researchers often use PLD to explore new material systems for applications in electronics, photonics, and quantum technologies. Its ability to grow complex oxides with high precision has made it a cornerstone in material science.
Metal-Organic Chemical Vapor Deposition (MOCVD) in Semiconductor Fabrication
Metal-Organic Chemical Vapor Deposition (MOCVD) has become the most popular method for epitaxial growth, especially in semiconductor fabrication. This technique involves introducing metal-organic precursors and reactive gases into a chamber. These gases react on the heated substrate, forming a crystalline layer.
MOCVD offers a balance between precision and cost-effectiveness. It is widely used in industries to produce high-quality semiconductor materials, such as gallium nitride (GaN) and silicon carbide (SiC). These materials are essential for devices like LEDs, laser diodes, and power electronics. The scalability of MOCVD makes it a preferred choice for mass production, ensuring consistent quality across large batches.
“MOCVD combines precision with scalability, making it the go-to method for producing high-performance semiconductor materials.”
Each of these methods brings unique strengths to the table. MBE excels in precision, PLD shines in versatility, and MOCVD leads in scalability. Together, they form the backbone of advanced epitaxial growth technology, driving innovation in applied materials epitaxy.
2D Material-Based Epitaxy for Next-Generation Devices
2D material-based epitaxy has emerged as a groundbreaking approach in the development of next-generation devices. This method leverages the unique properties of two-dimensional materials, such as graphene and hexagonal boron nitride (h-BN), to create high-performance crystalline films. These materials, with their atomically thin structures, offer exceptional flexibility and compatibility with various substrates, making them ideal for advanced applications.
Why 2D Materials Matter
Two-dimensional materials stand out due to their remarkable electrical, mechanical, and thermal properties. For instance, graphene exhibits extraordinary electrical conductivity and mechanical strength, while h-BN provides excellent thermal stability and insulation. These characteristics make 2D materials highly desirable for epitaxial growth, especially in fields like electronics, photonics, and quantum computing.
By using 2D materials as buffer layers, researchers can overcome traditional challenges in epitaxial growth, such as lattice mismatch. This flexibility allows the growth of high-quality films on substrates that were previously incompatible. The result is a new class of hybrid material systems with tailored properties, opening doors to innovative device designs.
Applications in Advanced Technologies
2D material-based epitaxy plays a pivotal role in several cutting-edge technologies:
- Quantum Computing: The precise control offered by 2D materials enables the creation of quantum dots and other structures essential for quantum information systems.
- Flexible Electronics: The mechanical flexibility of 2D materials supports the development of bendable and stretchable devices, such as wearable sensors and foldable displays.
- High-Efficiency Photonics: 2D materials enhance the performance of optoelectronic devices, including lasers, LEDs, and solar cells, by improving light absorption and emission properties.
Techniques Driving Innovation
Several epitaxial growth techniques, such as Molecular Beam Epitaxy (MBE) and Metal-Organic Chemical Vapor Deposition (MOCVD), have been adapted to incorporate 2D materials. MBE offers atomic-level precision, allowing researchers to fine-tune the thickness and composition of the grown layers. MOCVD, on the other hand, provides a cost-effective solution for large-scale production, making it suitable for industrial applications.
“MOCVD is the most popular method for epitaxial growth, balancing precision and scalability for high-performance semiconductor materials.”
A Future of Possibilities
The integration of 2D materials into epitaxial growth processes represents a significant leap forward in material science. By combining the strengths of traditional epitaxy with the versatility of 2D materials, researchers are paving the way for devices that were once thought impossible. From quantum computing to flexible electronics, the potential applications are vast and transformative.
As industries continue to explore the capabilities of 2D material-based epitaxy, the technology promises to redefine the boundaries of innovation. This exciting field holds the key to unlocking the next generation of devices, driving progress across multiple sectors.
Applications of Epitaxial Growth in Applied Materials
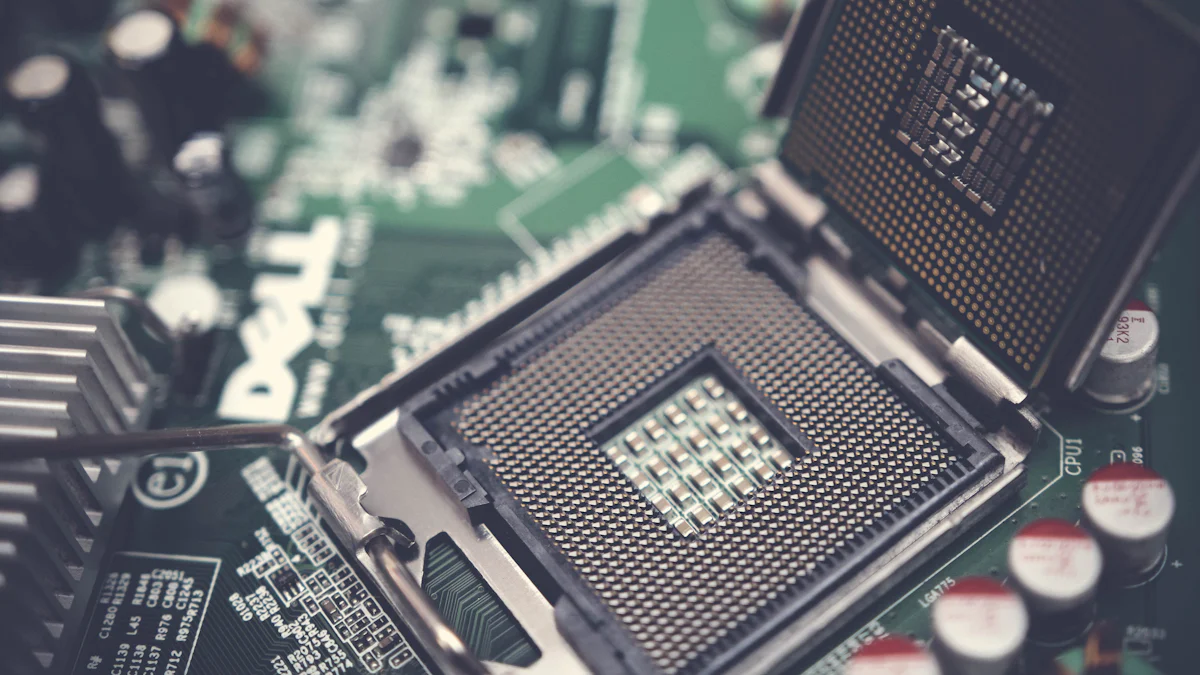
Epitaxial growth technology has revolutionized various industries by enabling the creation of high-quality crystalline materials. Its applications span across electronics, optoelectronics, and emerging fields, driving innovation and enhancing device performance. Let’s explore how this technology is shaping these areas.
Electronics: Enhancing Semiconductor Devices
Epitaxial growth plays a critical role in advancing semiconductor devices. By creating defect-free crystalline layers, it improves the electrical properties of semiconductors. This precision ensures that devices like transistors, diodes, and integrated circuits operate efficiently. For instance, silicon-based semiconductors benefit from epitaxial layers that enhance conductivity and reduce energy loss.
The ability to engineer materials at the atomic level allows manufacturers to develop smaller, faster, and more reliable electronic components. This advancement supports the growing demand for high-performance devices in consumer electronics, automotive systems, and telecommunications. Epitaxial growth also enables the integration of new materials, such as gallium nitride (GaN) and silicon carbide (SiC), which are essential for power electronics and high-frequency applications.
Optoelectronics: LEDs, Laser Diodes, and Solar Cells
In optoelectronics, epitaxial growth technology has transformed the production of light-emitting diodes (LEDs), laser diodes, and solar cells. These devices rely on high-quality crystalline layers to achieve optimal performance. For example, LEDs use epitaxially grown materials like GaN to produce bright, energy-efficient light. The precise control over material properties ensures consistent color and brightness, making LEDs ideal for displays, lighting, and automotive applications.
Laser diodes, used in communication systems and medical devices, also benefit from epitaxial growth. The technology enables the creation of thin films with tailored optical properties, ensuring high efficiency and reliability. In solar cells, epitaxial growth enhances light absorption and energy conversion. This improvement boosts the efficiency of photovoltaic systems, supporting the global shift toward renewable energy.
Emerging Fields: Quantum Computing and Advanced Sensors
Epitaxial growth is paving the way for breakthroughs in quantum computing and advanced sensors. In quantum computing, the technology enables the fabrication of quantum dots, qubits, and other structures essential for quantum information processing. The precise control over material properties ensures the stability and performance of these components, which are crucial for developing scalable quantum systems.
Advanced sensors, used in fields like healthcare, environmental monitoring, and defense, also benefit from epitaxial growth. The technology allows for the creation of materials with enhanced sensitivity and selectivity. For instance, epitaxially grown 2D materials, such as graphene, enable the development of sensors capable of detecting minute changes in temperature, pressure, or chemical composition.
“Epitaxy of 2D materials growth is crucial for the synthesis of wafer-scale single crystals, enabling innovations in advanced technologies.”
The integration of epitaxial growth into these emerging fields highlights its potential to drive future innovations. By combining traditional methods with cutting-edge techniques, applied materials epitaxy continues to expand the possibilities for next-generation devices.
Challenges in Applied Materials Epitaxy
Thermal Misfit and Strain Management
Thermal misfit presents a significant challenge in epitaxial growth. When the substrate and the deposited layer expand or contract at different rates due to temperature changes, stress builds up. This mismatch can lead to strain, which may cause cracks, dislocations, or even complete failure of the material. Managing this strain is critical for ensuring the structural integrity of the crystalline layers.
To address this issue, researchers focus on selecting substrates with thermal expansion properties that closely match the epitaxial layer. For example, in semiconductor fabrication, materials like silicon carbide (SiC) are often chosen for their compatibility with high-performance films. Additionally, advanced techniques, such as introducing buffer layers, help absorb the strain and reduce the risk of defects. These methods ensure that the final product maintains its quality and performance, even under demanding conditions.
“Reducing the thermal budget of epitaxial thin film growth remains a significant challenge, especially in industries like electronics where performance and cost are critical factors.”
Strain management also plays a vital role in enhancing the electrical and mechanical properties of the material. By minimizing defects caused by thermal misfit, manufacturers can produce devices with improved efficiency and reliability. This is particularly important in applications like micro-LED technology, where even minor imperfections can impact brightness and color purity.
Material Stability and Defect Reduction
Material stability is another hurdle in applied materials epitaxy. During the growth process, maintaining the stability of the crystalline structure is essential for achieving high-quality films. However, factors like contamination, uneven deposition, and lattice mismatches can introduce defects. These imperfections compromise the material’s performance and limit its application in advanced technologies.
To combat these challenges, epitaxial growth techniques have evolved to prioritize precision and control. Methods like Molecular Beam Epitaxy (MBE) and Metal-Organic Chemical Vapor Deposition (MOCVD) allow for atomic-level accuracy, reducing the likelihood of defects. For instance, MBE operates in an ultra-high vacuum environment, minimizing contamination and ensuring the purity of the grown layers.
Defect reduction is particularly crucial in industries like quantum computing and optoelectronics. In quantum computing, even the smallest defect can disrupt the delicate quantum states required for processing information. Similarly, in optoelectronic devices like LEDs and laser diodes, defects can affect light emission and energy efficiency. By refining epitaxial growth processes, researchers aim to create materials that meet the stringent requirements of these cutting-edge applications.
“Epitaxy plays a crucial role in growing crystals with specific orientations, enabling the creation of high-quality semiconductor wafers and optoelectronic devices.”
The demand for advanced electronic systems continues to rise, driving the need for defect-free semiconductor wafers. Epitaxial growth remains at the forefront of this effort, enabling the production of materials with exceptional stability and performance. Through ongoing innovation, the industry strives to overcome these challenges and unlock new possibilities in applied materials.
Opportunities for Innovation in Epitaxial Growth
Development of Hybrid Material Systems
Hybrid material systems represent a promising frontier in epitaxial growth. By combining different materials, researchers can create structures with unique properties that surpass the limitations of single-material systems. For instance, integrating two-dimensional (2D) materials like graphene with traditional semiconductors opens up possibilities for enhanced electrical conductivity and mechanical flexibility. These hybrid systems pave the way for advanced applications in electronics, photonics, and energy storage.
The ability to engineer hybrid materials also addresses challenges like thermal misfit and strain. Researchers can design buffer layers or intermediate materials to bridge mismatched lattices, reducing defects and improving stability. This approach not only enhances material performance but also expands the range of substrates suitable for epitaxial growth. Industries focused on quantum computing and flexible electronics stand to benefit significantly from these advancements.
“Hybrid material systems offer a pathway to overcome traditional limitations in epitaxial growth, enabling the creation of innovative devices with tailored properties.” – European-MRS Symposium
By exploring hybrid systems, scientists are unlocking new opportunities for innovation. These materials hold the potential to revolutionize industries by delivering unprecedented performance and versatility.
Integration of AI in Epitaxial Growth Processes
Artificial intelligence (AI) is transforming the way epitaxial growth processes are managed and optimized. Machine learning algorithms can analyze vast amounts of data from growth experiments, identifying patterns and predicting outcomes with remarkable accuracy. This capability allows researchers to fine-tune parameters like temperature, pressure, and deposition rates, ensuring consistent quality and efficiency.
AI also accelerates the discovery of new materials. By simulating growth conditions and predicting material properties, AI reduces the need for trial-and-error experiments. This approach saves time and resources while driving innovation in applied materials. For example, AI has been instrumental in identifying optimal conditions for growing defect-free films, addressing long-standing challenges in the field.
“The integration of AI into epitaxial growth processes has the potential to revolutionize material science, enabling faster and more efficient development of high-performance materials.”
Industries adopting AI-driven epitaxial growth gain a competitive edge by achieving higher precision and scalability. As AI technology continues to evolve, its role in material science will only grow, opening doors to groundbreaking advancements.
Scalable and Cost-Effective Growth Techniques
Scalability and cost-effectiveness remain critical goals in epitaxial growth technology. Traditional methods like Molecular Beam Epitaxy (MBE) offer exceptional precision but are often limited by high costs and low throughput. To address these challenges, researchers are developing innovative techniques that balance quality with affordability.
Metal-Organic Chemical Vapor Deposition (MOCVD) exemplifies this shift toward scalable solutions. Its ability to produce high-quality films at a lower cost makes it ideal for mass production. Advances in MOCVD technology have further improved its efficiency, enabling the growth of complex materials like gallium nitride (GaN) and silicon carbide (SiC) for industrial applications.
Another promising approach involves reducing the thermal budget of epitaxial growth. Lowering the temperature during the process minimizes energy consumption and prevents issues like electron tunneling, which can degrade device performance. This strategy not only cuts costs but also enhances the reliability of the final product.
“Reducing the thermal budget of epitaxial thin film growth has been a significant challenge, but it holds the key to achieving cost-effective and high-performance materials.” – IntechOpen Chapter
By prioritizing scalability and affordability, epitaxial growth technology is becoming more accessible to a wider range of industries. These advancements ensure that cutting-edge materials can meet the growing demand for high-performance devices without compromising quality.
Epitaxial growth technology has redefined material science by combining precision, innovation, and adaptability. Its principles, such as lattice matching and remote epitaxy, ensure the creation of high-quality crystalline layers. Advanced methods like Molecular Beam Epitaxy and Van der Waals epitaxy have enabled breakthroughs in semiconductors, optoelectronics, and quantum computing. Applied materials epitaxy continues to drive progress in modern industries, offering solutions for challenges like defect reduction and scalability. As hybrid approaches and AI integration advance, the future of epitaxial growth promises transformative possibilities for next-generation devices and sustainable technologies.
FAQ
What is epitaxial growth technology?
Epitaxial growth technology involves depositing a crystalline layer on a substrate, ensuring the layer aligns perfectly with the substrate’s crystal structure. This process creates high-quality materials with precise properties, essential for advanced applications like semiconductors and optoelectronics.
Why is lattice matching important in epitaxial growth?
Lattice matching ensures the crystal structures of the substrate and the deposited layer align seamlessly. This alignment minimizes defects, enhancing the material’s electrical, mechanical, and optical properties. Without proper lattice matching, the material may experience strain or instability, reducing its performance.
How does Van der Waals epitaxy differ from traditional methods?
Van der Waals epitaxy (vdWE) allows crystalline films to grow on two-dimensional (2D) materials without requiring lattice matching. This flexibility enables the creation of hybrid material systems, such as combining graphene with other materials. These systems open new possibilities for next-generation devices like quantum computers and flexible electronics.
What are the advantages of remote epitaxy?
Remote epitaxy uses a thin 2D-material buffer layer between the substrate and the growing film. This method allows the film to maintain its structural relationship with the substrate while remaining detachable. The key benefits include substrate reusability, cost reduction, and sustainable production processes.
Which industries benefit most from epitaxial growth technology?
Epitaxial growth technology impacts industries like electronics, optoelectronics, and renewable energy. It enhances semiconductor devices, improves LED and laser diode performance, and boosts solar cell efficiency. Emerging fields like quantum computing and advanced sensors also rely on this technology for innovation.
What makes Metal-Organic Chemical Vapor Deposition (MOCVD) popular?
MOCVD balances precision and scalability, making it ideal for industrial applications. It produces high-quality materials like gallium nitride (GaN) and silicon carbide (SiC), essential for LEDs, power electronics, and other devices. Its cost-effectiveness and ability to handle large-scale production make it a preferred choice.
Can epitaxial growth reduce production costs?
Yes, advanced methods like remote epitaxy, Van der Waals epitaxy, and layer transfer technologies significantly lower production costs. These techniques enable mass production of high-performance devices by improving material efficiency and reducing waste. For example, 2DLT transfer technology supports scalable manufacturing.
How does AI contribute to epitaxial growth processes?
Artificial intelligence optimizes epitaxial growth by analyzing data and predicting outcomes. It helps researchers fine-tune parameters like temperature and deposition rates, ensuring consistent quality. AI also accelerates material discovery, reducing trial-and-error experiments and driving innovation in applied materials.
What challenges does epitaxial growth face?
Epitaxial growth faces challenges like thermal misfit, strain management, and defect reduction. Thermal misfit occurs when the substrate and layer expand at different rates, causing stress. Advanced techniques, such as buffer layers and precise growth methods, address these issues to ensure material stability.
What is the future of epitaxial growth technology?
The future of epitaxial growth lies in hybrid material systems, AI integration, and scalable techniques. Combining 2D materials with traditional substrates unlocks new possibilities for device innovation. AI-driven processes enhance precision and efficiency, while cost-effective methods make high-performance materials accessible to more industries.