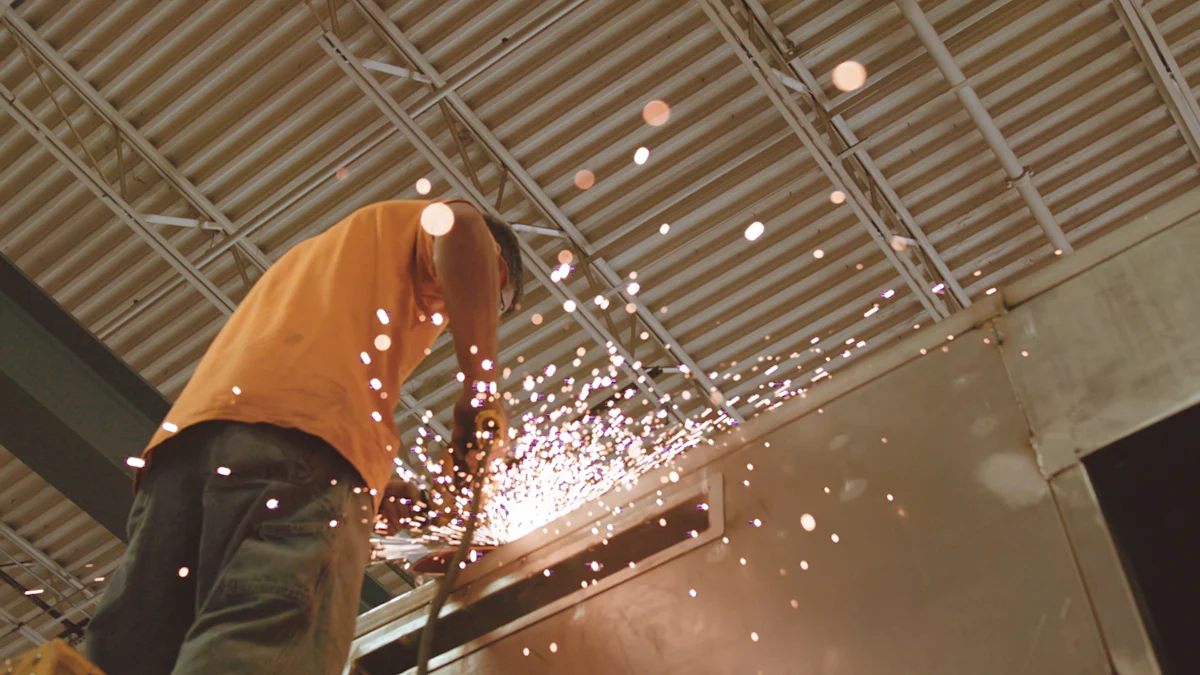
Siliziumkarbid (SiC)-Wafer spielen eine zentrale Rolle bei der modernen Leistungselektronik. Die Herstellung von SiC-Wafern umfasst eine Reihe von komplizierten Prozessen, die jeweils für außergewöhnliche Qualität und Leistung ausgelegt sind. Vom Kristallwachstum bis zum Polieren sorgt jeder Schritt dafür, dass die SiC-Wafer die strengen Standards erfüllen, die für Halbleiteranwendungen erforderlich sind. Der globale SiC-Wafermarkt, geschätzt bei $750,5 Mio in 2023, wird in einem 14.81TP3 CAGR bis 2030, angetrieben durch steigende Nachfrage in Elektrofahrzeugen und erneuerbare Energien. Diese Fortschritte unterstreichen die Bedeutung von Präzision und Innovation bei der Herstellung von SiC-Wafern.
Wichtigste Erkenntnisse
- SiC-Wafer sind für die moderne Leistungselektronik unerlässlich und ermöglichen schnellere und effizientere Geräte in Anwendungen wie Elektrofahrzeuge und erneuerbare Energien.
- Die manufacturing process beinhaltet kritische schritte: kristallwachstum, schneiden, polieren, doping und strenge tests, um hohe qualität und leistung zu gewährleisten.
- Fortgeschrittene Techniken wie Physical Vapor Transport (PVT) werden zum Kristallwachstum verwendet, um hohe Reinheit und Minimierung von Defekten in SiC-Wafern zu gewährleisten.
- Die Automatisierung beim Schneiden und Polieren verbessert die Präzision, reduziert Materialabfälle und verbessert die Gesamtproduktionseffizienz.
- Innovations in dopingtechnologien, wie Ionenimplantation, ermöglichen eine präzise Kontrolle über die elektrischen Eigenschaften von SiC-Wafern, die sie für bestimmte Anwendungen anpassen.
- KI spielt eine entscheidende Rolle bei der Qualitätskontrolle, indem Vorhersageanalysen verwendet werden, um Fehler frühzeitig zu erkennen und die Einhaltung der Industriestandards zu gewährleisten.
- Die wachsende Nachfrage nach SiC-Wafern wird durch ihre überlegene Leistung in High-Power- und Hochfrequenz-Anwendungen angetrieben, was sie zu einem Eckpfeiler der zukünftigen Halbleitertechnologie macht.
Übersicht über SiC Wafer Manufacturing
Herstellung von SiC-Wafer beinhaltet eine reihe hochspezialisierter prozesse, die ihre eignung für fortgeschrittene halbleiteranwendungen gewährleisten.
Schlüsselrolle von SiC Wafers in Electronics
SiC-Wafer haben die Elektronikindustrie revolutioniert, indem es schnellere, effizientere Geräte entwickelt. Ihre einzigartigen Eigenschaften, wie hohe Wärmeleitfähigkeit und die Fähigkeit, bei erhöhten Temperaturen zu arbeiten, machen sie unverzichtbar in der Leistungselektronik, erneuerbaren Energiesystemen und Elektrofahrzeugen. Geräte wie Schottky-Dioden, Metalloxid-Halbleiter-Feldeffekttransistoren (MOSFETs) und Schalter verlassen sich auf diese Wafer für eine überlegene Leistung. Darüber hinaus sorgt ihre Fähigkeit, extreme Umgebungen zu bewältigen, für Zuverlässigkeit in kritischen Anwendungen, einschließlich Luft- und Industrieanlagen.
"Siliconcarbid ist aufgrund seiner überlegenen dielektrischen Durchschlagfeldstärke und Wärmeleitfähigkeit zum bevorzugten Substratmaterial für fortgeschrittene Halbleiter, insbesondere in der Leistungselektronik, geworden."
Zusammenfassung der Fertigungsschritte
Die Produktion SiC-Wafer mit mehreren sorgfältig kontrollierten Schritten. Jede Stufe trägt dazu bei, die für Halbleiteranwendungen erforderlichen Qualitätsstandards zu erreichen.
Kristallwachstum
Das Verfahren beginnt mit Kristallwachstum, wobei hochreine Siliziumkarbidkristalle gebildet werden. Techniken wie Physical Vapor Transport (PVT) werden häufig verwendet, um einkristalline SiC-Ingots zu wachsen. Dieser Schritt ist entscheidend für die Bestimmung der strukturellen Integrität und elektrischen Eigenschaften des Wafers.
Schneiden und Polieren
Nach dem Kristallwachstum wird das Ingot mit Präzisionsschneidwerkzeugen in dünne Wafer geschnitten. Der Schneidvorgang gewährleistet eine gleichmäßige Dicke über alle Wafer. Das Polieren folgt, wodurch eine fehlerfreie Oberfläche entsteht, die für nachfolgende Fertigungsschritte wesentlich ist. Diese Stufe verbessert die Glätte des Wafers und bereitet sie auf Doping und Testen vor.
Doping und Ionenimplantation
Die Dotierung führt spezifische Verunreinigungen in den Wafer ein, um seine elektrischen Eigenschaften zu verändern. Ionenimplantation, eine präzise Technik, sorgt für die kontrollierte Einführung dieser Verunreinigungen. Dieser Schritt schneidet die Leitfähigkeit des Wafers und ermöglicht seinen Einsatz in verschiedenen elektronischen Geräten.
Prüfung und Qualitätssicherung
Die Endphase beinhaltet strenge Tests und Qualitätssicherung. Elektrische und strukturelle Tests überprüfen die Einhaltung der Industriestandards des Wafers. Fortgeschrittene Prüfverfahren erfassen etwaige Mängel und stellen sicher, dass nur hochwertige Wafer zur Gerätefertigung gelangen.
Detaillierte Prozesse in SiC Wafer Fertigung
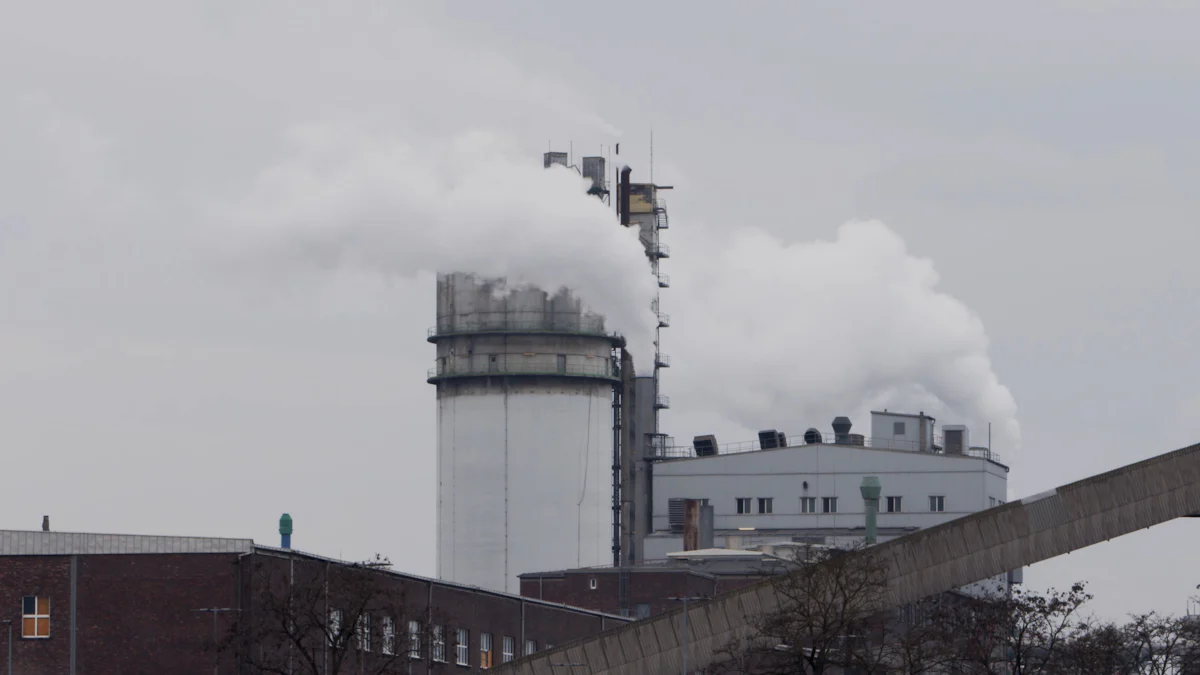
Kristallwachstum
Methoden wie physikalischer Dampftransport (PVT)
Hersteller verwenden in erster Linie die Körperlicher Vapor-Transport (PVT) verfahren zum anwachsen von hochreinen siliziumkarbidkristallen.
Das PVT-Verfahren ermöglicht eine präzise Steuerung der Wachstumsumgebung, einschließlich Temperatur, Druck und Gasfluss. Diese Faktoren beeinflussen die Bildung verschiedener Polytypen wie 4H-SiC und 6H-SiC, die in Halbleiteranwendungen weit verbreitet sind. Das PVT-Verfahren bleibt nach Studien der effektivste Ansatz für die Herstellung hochwertiger SiC-Kristalle aufgrund seiner Fähigkeit, Fehler zu minimieren und strukturelle Integrität zu gewährleisten.
Herausforderungen bei der Erzielung hochreiner Kristalle
Trotz seiner Effektivität stellt die Erzielung hochreiner SiC-Kristalle große Herausforderungen dar. Verunreinigungen im Quellmaterial oder Temperaturschwankungen können innerhalb der Kristallstruktur zu Defekten wie Mikrorohren oder Dislokationen führen. Diese Defekte können die elektrischen und mechanischen Eigenschaften der Wafer beeinträchtigen.
Forscher haben rechnerische Simulationen, wie die Modified Embedded Atom Method (MEAM) untersucht, um Fehlerbildung während des Kristallwachstums besser zu verstehen. Durch die Optimierung der Wachstumsbedingungen und die Verfeinerung des PVT-Prozesses wollen die Hersteller diese Unvollkommenheiten reduzieren und die Gesamtqualität von SiC-Wafern verbessern.
Schneiden und Polieren
Präzisionsschneiden für gleichmäßige Dicke
Nach dem Kristallwachstum erfährt der SiC-Ingot ein Slicing, um einzelne Wafer zu erzeugen. Präzisionsschneidwerkzeuge, oft mit diamantbeschichteten Messern ausgestattet, sorgen dafür, dass jeder Wafer eine gleichmäßige Dicke erreicht. Für nachfolgende Fertigungsschritte ist eine gleichbleibende Dickenkonsistenz kritisch, da sie die Leistung von Halbleiterbauelementen direkt beeinflusst.
Der Schneidvorgang erfordert fortschrittliche Maschinen, um Materialabfälle zu minimieren und Schäden an den Waferkanten zu verhindern. Die Automatisierung in dieser Phase hat deutlich verbesserte Effizienz und Genauigkeit, so dass Hersteller Wafer mit gleichbleibenden Abmessungen herstellen können.
Polieren für eine fehlerfreie Oberfläche
Hersteller verwenden chemisch-mechanisches Polieren (CMP) techniken, um eine fehlerfreie und ultraglättende oberfläche zu erreichen.
Eine polierte Oberfläche ist wesentlich, um eine optimale Leistung in Halbleiterbauelementen zu gewährleisten. Es reduziert die Wahrscheinlichkeit von Mängeln während der Dotierung und Prüfung, die Verbesserung der Zuverlässigkeit des Endproduktes. Das Polieren verbessert auch die Reflektivität des Wafers, was für bestimmte optische Anwendungen entscheidend ist.
Doping und Ionenimplantation
Steuerung elektrischer Eigenschaften durch Dotierung
Die Dotierung führt spezifische Verunreinigungen in den SiC-Wafer ein, um seine elektrischen Eigenschaften zu verändern. Dieser Prozess schneidet die Leitfähigkeit des Wafers und ermöglicht seinen Einsatz in verschiedenen elektronischen Geräten. So erzeugt z.B. Stickstoff n-Typ SiC, während Aluminium oder Bor p-Typ SiC produziert. Diese Variationen ermöglichen es den Herstellern, Wafer für bestimmte Anwendungen wie Leistungstransistoren oder Dioden anzupassen.
Techniken zur Einführung von Verunreinigungen
Ionenimplantation ist die präziseste Methode zur Dotierung von SiC-Wafern. Bei dieser Technik wird die Waferoberfläche mit hochenergetischen Ionen bombardiert und in das Kristallgitter eingebettet. Die Ionenimplantation bietet eine unvergleichliche Kontrolle über die Konzentration und Verteilung von Verunreinigungen und gewährleistet einheitliche elektrische Eigenschaften über den Wafer.
Nach der Implantation aktiviert ein Glühvorgang die Dotierstoffe und repariert Schäden, die während des Ionenbeschusses entstehen. Dieser Schritt stellt sicher, dass der Wafer die für die Halbleiterproduktion erforderlichen strengen Qualitätsstandards erfüllt.
Prüfung und Qualitätssicherung
Elektrische und strukturelle Prüfverfahren
Die Prüfung stellt sicher, dass SiC-Wafer die strengen Anforderungen von Halbleiteranwendungen erfüllen. Hersteller verwenden fortschrittliche elektrische und strukturelle Prüfverfahren, um die Leistung und Zuverlässigkeit der Wafer zu überprüfen. Elektrotests bewerten die Leitfähigkeit, den Widerstand und die Mobilität der Träger. Diese Parameter bestimmen die Eignung des Wafers für Hochleistungs- und Hochfrequenzgeräte. Techniken wie Kapazitäts-Spannung (C-V) Profilierung und Hall-Effektmessungen liefern präzise Daten über die elektrischen Eigenschaften des Wafers.
Die Strukturprüfung konzentriert sich auf die Erkennung von Fehlern und die Sicherstellung der physischen Integrität des Wafers. Zerstörungsfreie Methoden wie Röntgenbeugung (XRD) und Raman-Spektroskopie analysieren die Kristallstruktur und detektieren Dislokationen oder Mikrorohre. Die Rasterelektronenmikroskopie (SEM) bietet eine detaillierte Abbildung der Oberfläche des Wafers und zeigt Unvollkommenheiten, die die Geräteleistung beeinflussen könnten. Diese Prüfverfahren gewährleisten, dass nur Wafer mit optimalen elektrischen und strukturellen Eigenschaften auf die nächsten Produktionsstufen gelangen.
Sicherstellung der Einhaltung von Industriestandards
Die Einhaltung der Industriestandards ist bei der Herstellung von SiC-Wafer kritisch. Organisationen wie die Internationale Elektrotechnische Kommission (IEC) und die Semiconductor Equipment and Materials International (SEMI) setzen Benchmarks für Waferqualität und -leistung. Hersteller halten diese Standards, um Konsistenz und Zuverlässigkeit in allen Produkten zu gewährleisten.
Qualitätssicherungsteams implementieren strenge Inspektionsprotokolle, um die Einhaltung zu überprüfen. Automatisierte optische Inspektion (AOI) Systeme erkennen Oberflächenfehler mit hoher Präzision, während fortgeschrittene Messtechnik Werkzeuge Waferstärke, Planheit und Durchmesser messen. Statistische Prozesssteuerung (SPC)-Techniken überwachen Produktionsparameter, um sicherzustellen, dass jeder Wafer die erforderlichen Spezifikationen erfüllt. Durch strenge Einhaltung der Industriestandards garantieren die Hersteller die Herstellung hochwertiger SiC-Wafer, die für hochmoderne Halbleiteranwendungen geeignet sind.
Innovationen in SiC Wafer Fertigung
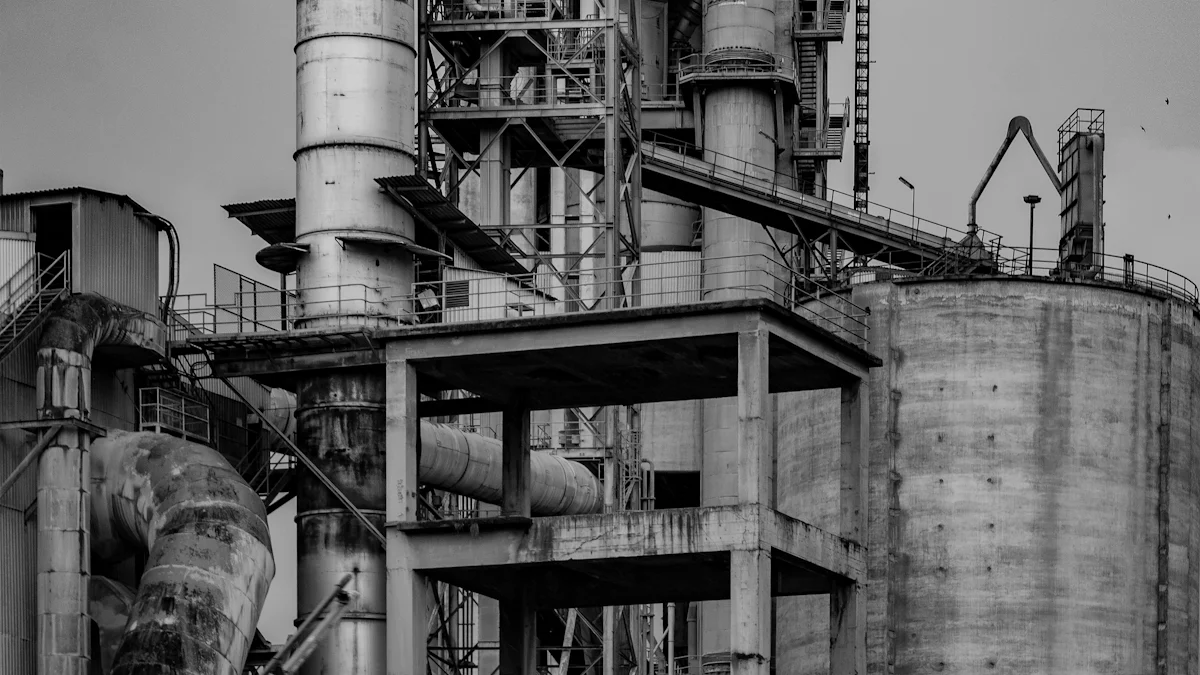
Advanced Crystal Growth Techniques
Schnellere Methoden mit weniger Defekten
Hersteller kontinuierlich verfeinern Kristallwachstum Techniken, um die Effizienz und Qualität der siliciumcarbid (SiC) Wafer. Neuere Fortschritte konzentrieren sich auf die Beschleunigung des Wachstumsprozesses bei der Minimierung von Mängeln.
Forscher erforschen auch alternative Methoden, darunter High-Temperature Chemical Vapor Deposition (HTCVD). Dieser Ansatz bietet schnellere Wachstumsraten und verbesserte Kristallgleichmäßigkeit. Durch die Bewältigung von Herausforderungen wie Defektdichte und Polytypstabilität sorgen diese Innovationen dafür, dass SiC-Wafer die strengen Anforderungen moderner Halbleiteranwendungen erfüllen.
"Die Abstufung von SiC-Wafern hängt stark von der Fehlerdichte und den elektrischen Eigenschaften ab, die ihre Leistung und Zuverlässigkeit in fortschrittlichen Geräten direkt beeinflussen."
Automatisierung in Schneiden und Polieren
Verbesserte Präzision und reduzierte Materialabfälle
Diamant-beschichtete Klingen, geführt von fortgeschrittene roboter, sicherzustellen, gleichmäßigkeit über alle wafer.
Polierprozesse profitieren auch von der Automatisierung. Chemikalienmechanische Poliersysteme (CMP) enthalten nun Echtzeitüberwachungs- und Rückkopplungsmechanismen. Diese Systeme passen Polierparameter dynamisch an und sorgen für fehlerfreie Oberflächen. Automatisierung verbessert nicht nur die Effizienz, sondern minimiert auch den menschlichen Fehler, wodurch hochwertige Wafer und reduzierte Produktionskosten entstehen.
KI in der Qualitätskontrolle
Prädiktive Analytik zur Defekterkennung
Künstliche Intelligenz (KI) spielt eine transformative Rolle in der Qualitätskontrolle für die Herstellung von SiC-Wafer. Machine Learning Algorithmen analysieren umfangreiche Datensätze aus Test- und Inspektionsprozessen. Diese Algorithmen identifizieren Muster und prognostizieren mögliche Fehler, bevor sie auftreten. Durch den Einsatz von Prädiktionsanalysen können die Hersteller Probleme frühzeitig lösen, Abfall reduzieren und die Produktqualität insgesamt verbessern.
KI-betriebene Systeme verbessern auch die Defekterkennung bei strukturellen und elektrischen Tests. Automatisierte optische Inspektion (AOI) Werkzeuge, mit KI ausgestattet, erkennen Oberflächenunregelmäßigkeiten mit unvergleichlicher Genauigkeit. Diese Systeme klassifizieren Fehler basierend auf Schwere und ermöglichen gezielte Korrekturmaßnahmen. Die Integration von AI sorgt dafür, dass SiC-Wafer die Industriestandards konsequent erfüllen und ihre Zuverlässigkeit in kritischen Anwendungen verstärken.
Neue Dopingtechnologien
Verbesserte Kontrolle über elektrische Eigenschaften
Fortschritte in Doping-Technologien haben die Fähigkeit revolutioniert, die elektrischen Eigenschaften von Siliziumkarbid (SiC)-Wafern zu feinabstimmen. Diese Innovationen ermöglichen es den Herstellern, eine präzise Kontrolle über die Leitfähigkeit zu erreichen und sicherzustellen, dass Wafer die spezifischen Anforderungen an Hochleistungshalbleitergeräte erfüllen.
Einer der bedeutendsten Durchbrüche ist die Entwicklung fortschrittlicher Ionenimplantationstechniken. Dieses Verfahren führt Verunreinigungen in das SiC-Kristallgitter mit unvergleichlicher Genauigkeit ein. Durch sorgfältige Steuerung der Energie und Dosierung von implantierten Ionen können Hersteller einheitliche Dotierungsprofile über den Wafer erreichen. Diese Präzision minimiert Schwankungen der elektrischen Eigenschaften, die für Anwendungen wie Leistungstransistoren und Dioden kritisch sind.
"Die Abstufung von Silizium-Carbide (SiC)-Wafern hängt stark von der Fehlerdichte und elektrischen Eigenschaften ab, die ihre Leistung und Zuverlässigkeit in fortschrittlichen Geräten direkt beeinflussen."
Neben der Ionenimplantation erforschen Forscher alternative Dotierungsmethoden, wie Plasmatauchionimplantation (PIII). Diese Technik bietet einen effizienteren Weg, um Verunreinigungen einzuführen, die Bearbeitungszeit zu reduzieren und gleichzeitig eine hohe Genauigkeit zu gewährleisten. PIII minimiert auch die Beschädigung der Waferoberfläche, bewahrt ihre strukturelle Integrität und verbessert die Gesamtleistung der Geräte.
Eine weitere Innovation beinhaltet die Verwendung von computergestützter Modellierung zur Optimierung von Doping-Prozessen. Durch die Simulation des Verhaltens von Verunreinigungen innerhalb des SiC-Gitters können Ingenieure die Ergebnisse verschiedener Dotierstrategien vorhersagen. Dieser Ansatz ermöglicht die Verfeinerung von Techniken, was zu Wafern mit überlegenen elektrischen Eigenschaften und weniger Defekten führt.
Diese Fortschritte in Doping-Technologien verbessern nicht nur die Qualität von SiC-Wafern, sondern erweitern auch ihre potenziellen Anwendungen. Eine verbesserte Kontrolle über elektrische Eigenschaften sorgt dafür, dass diese Wafer die anspruchsvollen Anforderungen der Stromelektronik, der erneuerbaren Energiesysteme und der Elektrofahrzeuge der nächsten Generation unterstützen können.
Vorteile von SiC Wafer Fertigungsprozessen
Qualität und Qualität
Höhere Reinheit und weniger Mängel
Die Herstellungsverfahren für Siliziumcarbid (SiC)-Wafer priorisieren die Erzielung außergewöhnlicher Reinheit und Minimierung von Defekten. Hochreine Materialien bilden die grundlage dieser wafer, da verunreinigungen ihre leistung in halbleiteranwendungen deutlich abbauen können.
Polierverfahren, einschließlich chemisch-mechanischem Polieren (CMP), verbessern die Oberflächenqualität durch Beseitigung von Unvollkommenheiten. Diese Schritte führen zusammen zu Wafern mit weniger Defekten, was eine verbesserte Geräteleistung und Langlebigkeit bedeutet. Wie Experten von Mersen betont: "Die Verwendung von hochreinen Materialien ist entscheidend, da Verunreinigungen die Leistung des Halbleiters erheblich beeinflussen können." Dieser Fokus auf Reinheit und Präzision sorgt dafür, dass SiC-Wafer die hohen Anforderungen der modernen Elektronik erfüllen.
Kosteneffizienz
Reduzierte Produktionskosten und Materialabfälle
SiC-Waferherstellungsprozesse beinhalten Innovationen, die die Ressourcennutzung optimieren und abfall reduzieren.
Darüber hinaus verwenden die Hersteller statistische Verfahren zur Kontrolle und Verfeinerung der Produktionsparameter. Dieser Ansatz reduziert die Variabilität und erhöht die Effizienz. Durch die Integration dieser kostensparenden Maßnahmen können Hersteller hochwertige Wafer zu einem geringeren Aufwand produzieren, wodurch SiC Wafer für weit verbreitete Anwendungen zugänglicher werden.
Enhanced Performance
Schnellere Produktionszyklen und bessere Geräteleistung
Innovationen in der SiC-Waferherstellung haben deutlich beschleunigte Produktionszyklen. Fortgeschrittene Kristallwachstumstechniken wie modifizierte PVT und High-Temperature Chemical Vapor Deposition (HTCVD), ermöglichen eine schnellere Ingotbildung ohne Qualitätseinbußen. Die Automatisierung beim Polieren und Schneiden beschleunigt den Produktionsprozess weiter und gewährleistet eine rechtzeitige Lieferung von Wafern an die Anforderungen der Industrie.
Die überlegenen elektrischen und thermischen Eigenschaften von SiC-Wafern verbessern die Leistung von Halbleiterbauelementen. Diese Wafer unterstützen hochleistungsfähige und hochfrequente Anwendungen, was sie in Elektrofahrzeugen, erneuerbaren Energiesystemen und Luft- und Raumfahrttechnologien unverzichtbar macht. Wie Experten von Mersen "Die Zukunft liegt in der Verbesserung der Fähigkeiten der epitaktischen Schichtung, insbesondere in Siliziumkarbid (SiC) Anwendungen." Dieses Engagement für Innovation stellt sicher, dass SiC-Wafer an der Spitze der Halbleitertechnologie bleiben.
Die Herstellung von SiC-Wafer basiert auf einer Reihe von fortschrittlichen Prozessen, einschließlich Kristallwachstum, Schneiden, Polieren, Doping und Tests. Diese Schritte gewährleisten die Herstellung von Wafern mit außergewöhnlicher Qualität und Leistung. Kontinuierliche Innovationen, wie selektive Dotierungstechniken wie Ionenimplantation, verbessern Effizienz und reduzieren Defekte. Da sich Branchen wie Elektrofahrzeuge und erneuerbare Energien ausweiten, wächst die Nachfrage nach SiC-Wafern rapide. Die Fortschritte in der Fertigung werden die Zukunft der Leistungselektronik prägen und SiC-Wafer als Eckpfeiler moderner Halbleitertechnologie verfestigen.
FAQ
Was macht SiC-Wafer den traditionellen Silizium-Wafern überlegen?
SiC-Wafer überlagern traditionelle Silizium-Wafer in mehreren Schlüsselbereichen. Sie weisen eine höhere Wärmeleitfähigkeit auf, so dass Geräte effizient bei erhöhten Temperaturen arbeiten können. Ihre überlegene dielektrische Durchschlagsfestigkeit ermöglicht die Schaffung schnellerer und zuverlässiger Leistungsgeräte. Diese Eigenschaften machen SiC-Wafer ideal für Anwendungen in Elektrofahrzeugen, Erneuerbare Energien und Hochfrequenzelektronik.
"Siliconcarbid-Wafer revolutionieren die Leistungselektronik, indem sie unübertroffene Effizienz und Leistung gegenüber Silizium bieten."
Warum werden SiC-Wafer für die Stromerzeugung bevorzugt?
SiC-Wafer ermöglichen die Herstellung von Leistungsgeräten, die bei höheren Spannungen und Temperaturen arbeiten. Ihre Fähigkeit, extreme Bedingungen zu handhaben, sorgt für Zuverlässigkeit in anspruchsvollen Anwendungen. Geräte mit SiC-Wafern, wie MOSFETs und Schottky-Dioden, liefern schnellere Schaltgeschwindigkeiten und reduzierte Energieverluste, was sie in Industrien wie Luft- und Raumfahrt und Automotive unverzichtbar macht.
Wie werden die Kosten für die Herstellung von SiC-Wafern mit Silizium-Wafern verglichen?
Die Herstellung von SiC-Wafern erfordert höhere Kosten durch die Komplexität von Prozessen wie Kristallwachstum und Polieren. Die fortschrittlichen Techniken, die erforderlich sind, um hochreine SiC-Kristalle zu erreichen, tragen zu diesen Kosten bei. Die langfristigen Vorteile, einschließlich verbesserter Geräteleistung und Energieeffizienz, überwiegen jedoch oft die anfängliche Investition.
Was sind die wichtigsten Schritte bei der Herstellung von SiC-Wafer?
Der Herstellungsprozess umfasst mehrere kritische Schritte: ANHANG. Kristallwachstum: Bildung von hochreinen SiC-Kristallen mit Methoden wie CVD SiC coating.
- Kristallwachstum: Bildung von hochreinen SiC-Kristallen mit Methoden wie Physical Vapor Transport (PVT).
- Schneiden und Polieren: Präzisionsschneiden und Polieren, um eine gleichmäßige Dicke und eine fehlerfreie Oberfläche zu erreichen.
- Doping und Ionenimplantation: Einführung von Verunreinigungen zu maßgeschneiderten elektrischen Eigenschaften.
- Prüfung und Qualitätssicherung: strenge Inspektionen, um die Einhaltung der Industriestandards zu gewährleisten.
Jeder Schritt gewährleistet die Herstellung von Wafern, die den strengen Anforderungen von Halbleiteranwendungen entsprechen.
Wie tragen SiC-Wafer zu erneuerbaren Energiesystemen bei?
SiC-Wafer verbessern die Effizienz und Zuverlässigkeit von erneuerbaren Energiesystemen. Ihre Fähigkeit, hohe Spannungen und Temperaturen zu handhaben, macht sie ideal für Wechselrichter in Solar- und Windenergieanwendungen. Durch die Reduzierung von Energieverlusten verbessern SiC-basierte Geräte die Gesamtleistung dieser Systeme und unterstützen den globalen Übergang zu nachhaltiger Energie.
Welche Herausforderungen gibt es bei der Herstellung von SiC-Wafer?
Herstellung SiC-Wafer stellen Herausforderungen wie die Erzielung von hochreinen Kristallen und die Minimierung von Defekten wie Mikrorohren und Dislokationen. Diese Unvollkommenheiten können die elektrischen und mechanischen Eigenschaften der Wafer beeinflussen. Fortgeschrittene Techniken, einschließlich computergestützter Modellierung und optimierten Wachstumsbedingungen, helfen Herstellern, diese Hindernisse zu überwinden.
Wie verbessert die Automatisierung die SiC-Waferproduktion?
Automatisierung verbessert Präzision und Effizienz bei Schneid- und Polierprozessen. Automatisierte Systeme mit diamantbeschichteten Werkzeugen sorgen für gleichbleibende Waferstärke und minimale Materialabfälle. Die Echtzeitüberwachung beim Polieren verbessert die Oberflächengüte, reduziert Fehler und Produktionskosten.
Welche Rolle spielt KI in SiC Wafer Qualitätskontrolle?
Künstliche Intelligenz verwandelt die Qualitätskontrolle, indem sie prädiktive Analytik und fortschrittliche Defekterkennung ermöglicht. Machine Learning Algorithmen analysieren Daten von Testprozessen, um Muster zu identifizieren und potenzielle Probleme vorherzusagen. KI-betriebene Inspektionswerkzeuge erfassen Oberflächenunregelmäßigkeiten mit außergewöhnlicher Genauigkeit, so dass nur hochwertige Wafer zur Gerätefertigung gelangen.
Sind SiC-Wafer umweltfreundlich?
SiC-Wafer tragen zur ökologischen Nachhaltigkeit bei, indem sie die Effizienz von Leistungsgeräten verbessern. Ihr Einsatz in Elektrofahrzeugen und erneuerbaren Energiesystemen reduziert den Energieverbrauch und die Treibhausgasemissionen. Obwohl ihre Herstellung einen hohen Energieverbrauch beinhaltet, überwiegen die langfristigen Vorteile bei Energieeinsparungen und reduzierten Umweltauswirkungen die ersten Kosten.
Welche Branchen profitieren am meisten von SiC-Wafern?
Industrien wie Automotive, Aerospace, Erneuerbare Energien und Telekommunikation profitieren maßgeblich von SiC-Wafern. Markttrends für Siliziumkarbid dummy wafer verbessern die wechselrichterleistung.