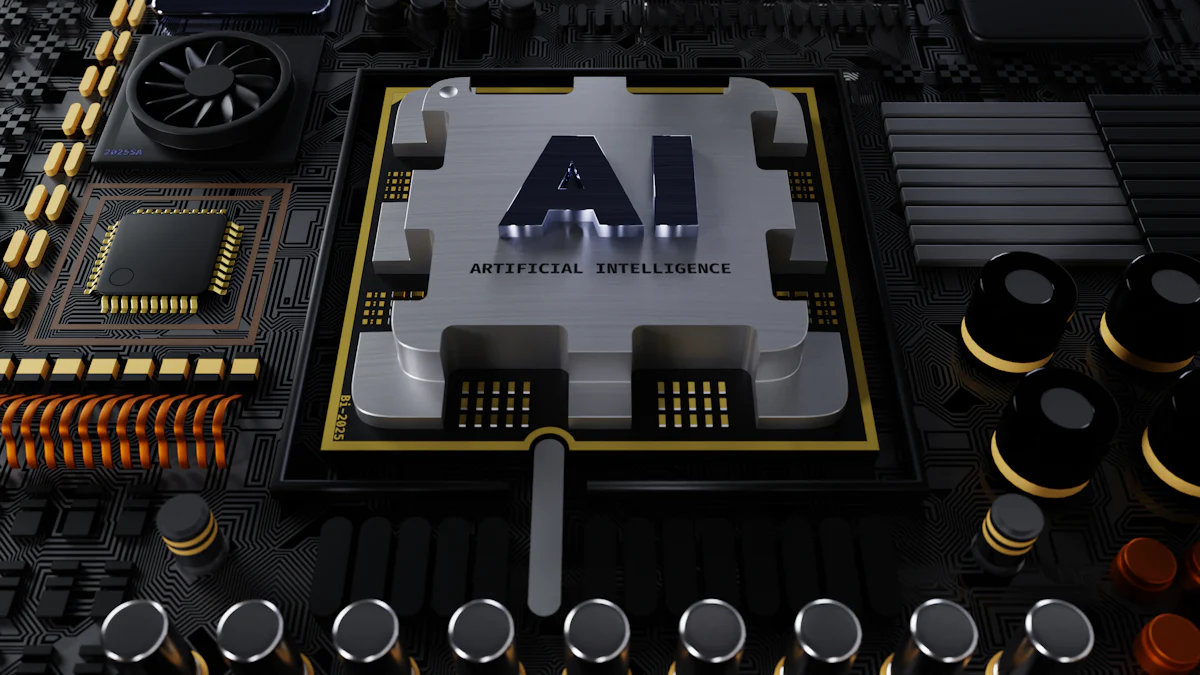
Silicon epitaxy susceptors play a pivotal role in the semiconductor industry, ensuring the precise thermal environment required for epitaxial growth. These components are indispensable for producing high-quality silicon wafers, which serve as the foundation for advanced semiconductor devices. The manufacturing process demands meticulous attention to detail, as even minor imperfections can compromise performance. By adhering to specific steps, manufacturers achieve the precision and reliability necessary for applications in sectors like automotive, aerospace, and renewable energy. The global market for these susceptors reflects their importance, with projections estimating growth from 2.5billionin2023to4.8 billion by 2032.
要点
- Choose high-purity materials like silicon, graphite, and silicon carbide to ensure thermal stability and chemical resistance, which are crucial for high-quality semiconductor performance.
- Utilize advanced shaping and machining techniques, such as diamond-tipped tools and abrasive machining, to achieve precise dimensions and enhance the structural integrity of susceptors.
- Implement thorough surface preparation methods, including chemical cleaning and polishing, to eliminate contaminants and ensure a defect-free surface for optimal epitaxial layer deposition.
- Control key factors during the epitaxial layer deposition process, such as growth pressure and temperature, to achieve uniform and high-quality layers essential for semiconductor devices.
- Conduct rigorous quality control and testing, including microscopic analysis and thermal conductivity testing, to verify the performance and reliability of silicon epitaxy susceptors before use.
Step 1: Material Selection for Silicon Epitaxy Susceptors
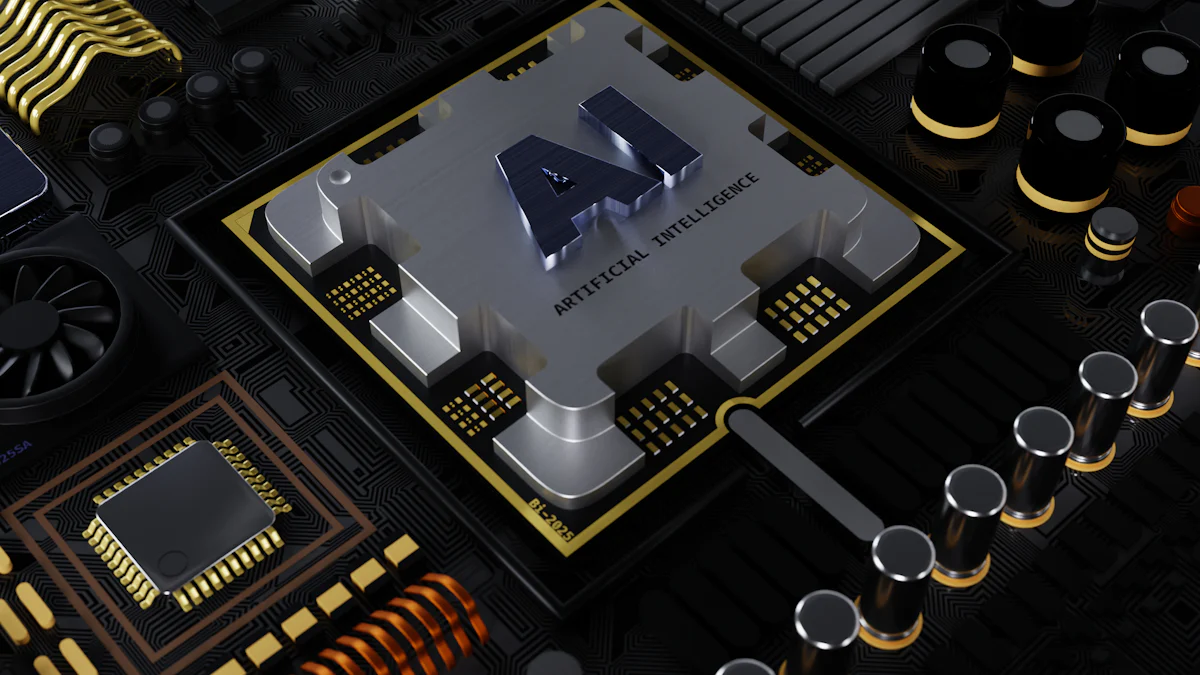
The foundation of a high-performing silicon epitaxy susceptor lies in the careful selection of materials. Manufacturers must prioritize materials that ensure thermal stability, chemical resistance, and uniform heat distribution. These properties directly influence the quality of the epitaxial layers and, consequently, the performance of semiconductor devices.
Importance of High-Purity Silicon
High-purity silicon serves as a cornerstone in the production of silicon epitaxy susceptors. Impurities in the material can lead to defects in the epitaxial layers, compromising the efficiency and reliability of the final semiconductor products. Manufacturers often source silicon with minimal contamination to meet the stringent requirements of the semiconductor industry.
"The performance of silicon epitaxy susceptors can significantly influence the quality of the epitaxial layers produced, impacting the overall efficiency of semiconductor devices."
In addition to purity, the silicon must exhibit excellent thermal conductivity to maintain consistent temperatures during the epitaxial growth process. This ensures that the silicon wafers receive uniform heating, which is critical for achieving defect-free layers.
Role of Additional Materials in Susceptor Manufacturing
While silicon remains a primary material, additional materials like graphite そして、 炭化ケイ素(SiC) play a vital role in enhancing the performance of silicon epitaxy susceptors. Graphite, known for its high thermal conductivity and stability at elevated temperatures, provides precise temperature control and uniform heat distribution. Its ability to withstand harsh chemical environments makes it a popular choice in the manufacturing process.
Silicon carbide, on the other hand, offers superior thermal conductivity and chemical resistance compared to graphite. The SiC coating on graphite susceptors enhances their durability, making them more resilient to thermal shock and reducing the risk of contamination. These characteristics make SiC-coated graphite susceptors an ideal choice for producing high-quality epitaxial layers.
-
Key Benefits of Graphite Susceptors:
- High thermal conductivity
- Stability at elevated temperatures
- 化学分解への抵抗
-
Advantages of Silicon Carbide (SiC) Susceptors:
- 高められた熱安定性
- Superior chemical resistance
- Reduced contamination risk
By combining these materials strategically, manufacturers can create silicon epitaxy susceptors that meet the demanding requirements of modern semiconductor applications. The choice of materials not only impacts the manufacturing process but also determines the overall efficiency and reliability of the susceptors in real-world applications.
Step 2: Shaping and Machining of Silicon Epitaxy Susceptors
The shaping and machining process transforms raw materials into precisely engineered silicon epitaxy susceptors. This stage demands exceptional accuracy to ensure the final product meets the stringent requirements of semiconductor manufacturing. Proper shaping and machining techniques enhance the susceptor’s structural integrity, thermal performance, and overall reliability.
Achieving Precision in Susceptor Shaping
Precision in shaping forms the backbone of high-quality silicon epitaxy susceptors. Manufacturers employ advanced methods to achieve the exact dimensions and geometries required for optimal performance. The process begins with cutting the raw material into the desired shape using diamond-tipped tools. These tools minimize material loss and ensure clean cuts, which are essential for maintaining uniformity.
Grinding and lapping follow the initial cutting phase. These techniques refine the surface and eliminate irregularities. Grinding, often performed with abrasive wheels, reduces surface roughness and prepares the susceptor for further processing. Lapping, a more delicate process, polishes the surface to achieve a mirror-like finish. This step is critical for ensuring defect-free epitaxial layer deposition.
"Ongoing research is focused on refining grinding techniques for SiC substrates to minimize surface roughness and subsurface damage." – Materials Science and Engineering Tumblr
Manufacturers must also account for thermal expansion during shaping. The susceptor’s design must withstand extreme temperatures without warping or cracking. This consideration ensures the susceptor maintains its structural integrity throughout the epitaxial growth process.
Tools and Techniques for Machining Silicon-Based Materials
Machining silicon-based materials, such as silicon carbide (SiC) and graphite, requires specialized tools and techniques. These materials exhibit high hardness and brittleness, making them challenging to machine. Diamond-coated tools are commonly used due to their ability to cut through hard surfaces with precision.
Abrasive machining plays a pivotal role in shaping silicon carbide susceptors. This technique uses abrasive particles to remove material and achieve the desired shape. It minimizes micro-cracks and subsurface damage, which could compromise the susceptor’s performance. Advanced abrasive machining technologies continue to evolve, offering improved efficiency and accuracy.
"Abrasive machining technology of SiC ceramic composites has been extensively reviewed to enhance manufacturing processes." – PubMed
In addition to traditional methods, manufacturers explore innovative techniques like microwave annealing. This method uses a SiC-alumina susceptor to recrystallize silicon substrates and activate dopants. It enhances the material’s properties, making it more suitable for high-performance applications.
"Microwave annealing with a SiC-alumina susceptor improves Si recrystallization and dopant activation." – AIP Journals
The combination of precise shaping and advanced machining techniques ensures that silicon epitaxy susceptors meet the rigorous demands of semiconductor production. These processes contribute to the creation of reliable and efficient components, which play a critical role in advancing modern technology.
Step 3: Surface Preparation of Silicon Epitaxy Susceptors
Surface preparation plays a crucial role in ensuring the performance and reliability of a silicon epitaxy susceptor. This step eliminates contaminants and imperfections, creating an ideal surface for epitaxial layer deposition. Proper cleaning and polishing techniques enhance the susceptor’s functionality, reducing the risk of defects during semiconductor manufacturing.
Cleaning Processes for Contaminant Removal
Cleaning removes impurities that can compromise the quality of the epitaxial layers. Manufacturers employ a series of cleaning processes to achieve a contaminant-free surface. These processes often include chemical cleaning, ultrasonic cleaning, and plasma cleaning.
Chemical cleaning uses specialized solutions to dissolve and remove organic and inorganic contaminants. For instance, hydrofluoric acid solutions effectively eliminate oxide layers and residues from the susceptor’s surface. This method ensures a clean and reactive surface, essential for subsequent polishing and deposition steps.
Ultrasonic cleaning introduces high-frequency sound waves into a liquid cleaning solution. These waves generate microscopic bubbles that collapse near the surface, dislodging particles and contaminants. This technique is particularly effective for removing fine debris from intricate susceptor geometries.
Plasma cleaning, another advanced method, utilizes ionized gas to break down and remove surface contaminants. This process not only cleans but also activates the surface, improving adhesion during epitaxial layer deposition. Plasma cleaning is especially beneficial for silicon carbide-coated susceptors, as it enhances their chemical compatibility with silicon wafers.
"A prepared surface behind, or alternatively, dry etching the silicon carbide surface" – Method of post-deposition treatment for silicon oxide film
By combining these cleaning techniques, manufacturers ensure that the susceptor surface is free from contaminants, enabling high-quality epitaxial growth.
Polishing Techniques for a Defect-Free Surface
Polishing refines the susceptor’s surface, eliminating irregularities and achieving the required smoothness. This step is critical for preventing defects such as warping, slip, or adhesion issues during the epitaxial growth process.
Mechanical polishing involves the use of abrasive materials to remove surface imperfections. Diamond abrasives are commonly used due to their hardness and precision. This method reduces surface roughness and prepares the susceptor for further processing.
Chemical-mechanical polishing (CMP) combines mechanical abrasion with chemical reactions to achieve a planar and defect-free surface. CMP is particularly effective for silicon carbide susceptors, as it minimizes micro-cracks and subsurface damage. This technique ensures uniformity, which is vital for consistent thermal performance.
"Polishing the surfaces of semiconductor wafers pursues the goal of removing material from the surfaces…to form a planar surface that is as uniform as possible." – Method for polishing semiconductor wafers
Finishing polish addresses any remaining surface irregularities. This step controls the height of protrusions to prevent defects like warping or adhesion. For instance, finishing polish ensures that protrusions on the susceptor’s surface do not exceed 5 μm, maintaining optimal performance during semiconductor manufacturing.
"The finishing polish may be given by either conventionally known machine polish or hand polish, as long as those surface roughness and protrusion height are achieved." – Method of post-deposition treatment for silicon oxide film
Through meticulous polishing, manufacturers create a defect-free surface that supports reliable and efficient epitaxial layer deposition. This attention to detail ensures that the silicon epitaxy susceptor meets the stringent demands of modern semiconductor applications.
Step 4: Epitaxial Layer Deposition on Silicon Epitaxy Susceptors
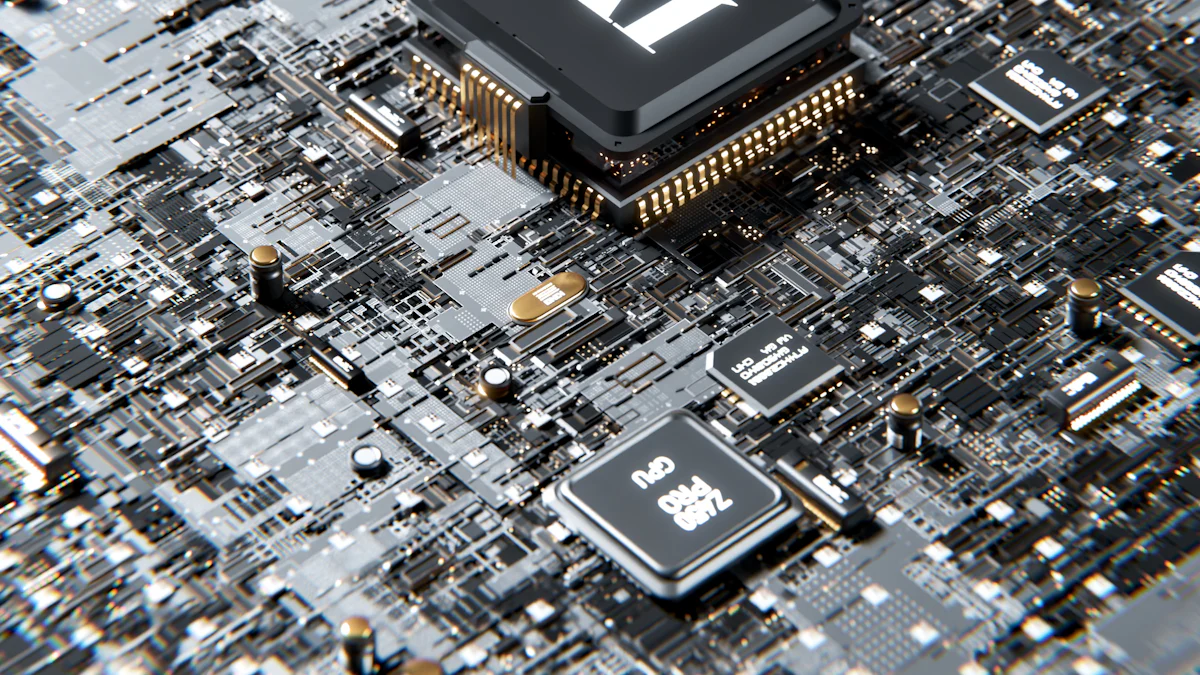
The epitaxial layer deposition process is a critical stage in the manufacturing of a silicon epitaxy susceptor. This step determines the structural and functional quality of the epitaxial layers, which directly impacts the performance of semiconductor devices. Manufacturers must carefully control various parameters to ensure the deposition process achieves the desired results.
Key Factors in the Epitaxy Process
Several factors influence the success of the epitaxial layer deposition process. Growth pressure, temperature, and the use of buffer layers play pivotal roles in achieving high-quality epitaxial layers. Each parameter requires precise calibration to optimize the deposition process.
-
Growth Pressure: The pressure within the deposition chamber significantly affects the crystal quality, thickness, and doping concentration of the epitaxial layers. Studies have shown that selecting the appropriate growth pressure enhances the uniformity and structural integrity of the layers. This adjustment ensures the epitaxial layers meet the stringent requirements of semiconductor applications.
-
温度制御: Maintaining a consistent temperature during deposition is essential for achieving uniform growth. Fluctuations in temperature can lead to defects, such as uneven thickness or poor adhesion. Advanced thermal management systems in the susceptor design help maintain stable temperatures, ensuring reliable deposition.
-
Buffer Layers: The inclusion of buffer layers between the susceptor and the epitaxial layer reduces lattice mismatch and stress. These layers act as a barrier, preventing impurities and defects from affecting the epitaxial layer. Research highlights that buffer layers improve surface roughness and enhance the overall quality of the deposited layers.
Scientific Insight: "Buffer layer growth reduces lattice mismatch and stress, improves quality and uniformity, and acts as a barrier for impurities and defects in the epitaxial layer."
By addressing these factors, manufacturers can optimize the epitaxy process, resulting in superior-quality layers that meet the demands of modern semiconductor technologies.
Ensuring Uniformity During Deposition
Uniformity in the epitaxial layer is crucial for the performance and reliability of semiconductor devices. Achieving this uniformity requires meticulous attention to the deposition environment and the properties of the susceptor.
-
Thermal Distribution: The silicon epitaxy susceptor must provide uniform heat distribution across its surface. Uneven heating can cause variations in layer thickness, leading to performance inconsistencies. Materials like silicon carbide, known for their excellent thermal conductivity, are often used to enhance thermal uniformity.
-
ガスの流れの動的: The flow of precursor gases within the deposition chamber influences the growth rate and uniformity of the epitaxial layer. Properly designed gas flow systems ensure even distribution of reactants, minimizing the risk of defects.
-
表面の準備: A well-prepared susceptor surface promotes uniform deposition. Cleaning and polishing techniques, as discussed earlier, eliminate contaminants and irregularities, creating an ideal foundation for the epitaxial layer.
Scientific Insight: "Buffer layer growth significantly influences the surface roughness, growth rate, and thickness uniformity of epitaxial layers."
Manufacturers also employ advanced monitoring systems to track the deposition process in real time. These systems detect anomalies and allow for immediate adjustments, ensuring consistent quality throughout the production cycle.
By focusing on these aspects, manufacturers achieve uniform epitaxial layers that enhance the efficiency and reliability of semiconductor devices. The precision and care invested in this step underscore the importance of the silicon epitaxy susceptor in advancing semiconductor technology.
Step 5: Quality Control and Testing of Silicon Epitaxy Susceptors
Quality control and testing ensure that every silicon epitaxy susceptor meets the rigorous standards required for semiconductor manufacturing. This step verifies the structural integrity, thermal performance, and mechanical reliability of the susceptors, guaranteeing their suitability for high-precision applications.
Inspection Methods for Surface and Structural Integrity
Manufacturers employ advanced inspection methods to assess the surface and structural integrity of silicon epitaxy susceptors. These methods identify defects, irregularities, or inconsistencies that could compromise performance during epitaxial growth.
-
Visual Inspection: High-resolution imaging systems detect surface imperfections such as scratches, cracks, or contamination. These systems provide detailed visual data, enabling manufacturers to address issues before further processing.
-
Microscopic Analysis: Scanning electron microscopy (SEM) examines the susceptor’s surface at a microscopic level. This technique reveals fine details, including surface roughness and micro-cracks, which are invisible to the naked eye.
-
Non-Destructive Testing (NDT): Techniques like ultrasonic testing and X-ray imaging evaluate the internal structure without damaging the susceptor. Ultrasonic waves detect voids or inclusions, while X-rays reveal internal defects that could weaken the susceptor under high temperatures.
-
Surface Profilometry: This method measures surface roughness and uniformity. A smooth and uniform surface is critical for achieving defect-free epitaxial layers. Profilometry ensures that the susceptor meets the required specifications for surface quality.
"Surface roughness and uniformity directly influence the quality of epitaxial layers, impacting the overall performance of semiconductor devices."
By combining these inspection methods, manufacturers ensure that each susceptor exhibits flawless surface and structural characteristics, essential for reliable semiconductor production.
Performance Testing for Thermal and Mechanical Reliability
Performance testing evaluates the thermal and mechanical properties of silicon epitaxy susceptors. These tests confirm that the susceptors can withstand the demanding conditions of epitaxial growth processes.
-
Thermal Conductivity Testing: Manufacturers measure the susceptor’s ability to distribute heat uniformly. Materials like silicon carbide excel in this area, providing consistent thermal performance. Uniform heat distribution prevents defects in the epitaxial layers, ensuring high-quality semiconductor wafers.
-
Thermal Shock Resistance: Susceptors undergo rapid heating and cooling cycles during epitaxial growth. Testing for thermal shock resistance ensures that the susceptor can endure these extreme temperature changes without cracking or warping.
-
Mechanical Strength Testing: Mechanical tests assess the susceptor’s ability to withstand physical stress. These tests measure parameters such as tensile strength, compressive strength, and fracture toughness. A robust susceptor resists deformation and maintains its structural integrity under operational loads.
-
Doping Uniformity Assessment: The choice of carbon sources, such as C3H8 and C2H4, influences doping uniformity. Testing confirms that the susceptor supports stable growth processes and achieves consistent doping concentrations. For example, C2H4 provides superior surface roughness and uniformity compared to C3H8, making it a preferred choice for achieving optimal results.
"C2H4 offers improved surface thickness uniformity and better doping uniformity, enhancing the overall quality of the epitaxial layers."
- Thermal Expansion Compatibility: Testing ensures that the susceptor’s thermal expansion matches the silicon wafers it supports. Mismatched thermal expansion can lead to stress and defects during epitaxial growth. Compatibility testing minimizes these risks, promoting reliable performance.
Through rigorous performance testing, manufacturers validate the reliability and efficiency of silicon epitaxy susceptors. These tests confirm that the susceptors meet the stringent demands of modern semiconductor applications, ensuring consistent quality and performance.
The manufacturing of a silicon epitaxy susceptor involves five critical steps, each contributing to its precision and reliability. From material selection to quality control, every stage ensures the production of high-performance components essential for semiconductor applications. Precision in shaping, surface preparation, and epitaxial layer deposition directly impacts the efficiency of these susceptors. Rigorous testing guarantees their durability under demanding conditions. These components play a pivotal role in advancing technologies like 5G, IoT, and AI by enabling the creation of superior semiconductor devices. Their importance underscores the need for continuous innovation in this field.
よくあるご質問
What are silicon epitaxial susceptors used for?
Silicon epitaxial susceptors play a vital role in the production of advanced semiconductor devices. They are essential for manufacturing high-efficiency solar cells, semiconductor components for electric vehicles, and autonomous driving technologies. These susceptors also support the development of cutting-edge technologies like 5G, IoT, and AI by enabling the creation of superior silicon wafers.
Why is the market for silicon epitaxial susceptors growing?
The demand for silicon epitaxial susceptors continues to rise due to advancements in semiconductor technology. The increasing adoption of silicon-based devices across industries drives this growth. High-quality silicon wafers, essential for modern semiconductor applications, further fuel the market’s expansion.
What materials are commonly used in silicon epitaxial susceptors?
Manufacturers often use materials like graphite and silicon carbide (SiC) in the production of silicon epitaxial susceptors. Graphite offers high thermal conductivity and stability, while SiC provides superior thermal resistance and durability. These materials ensure optimal performance during the epitaxial growth process.
How does surface preparation impact susceptor performance?
Surface preparation eliminates contaminants and imperfections, creating an ideal foundation for epitaxial layer deposition. Cleaning methods, such as chemical and plasma cleaning, remove impurities. Polishing techniques refine the surface, ensuring defect-free layers and enhancing the susceptor’s reliability.
What factors influence the epitaxial layer deposition process?
Several factors, including growth pressure, temperature control, and the use of buffer layers, significantly impact the epitaxial layer deposition process. Proper calibration of these parameters ensures uniformity, structural integrity, and high-quality epitaxial layers.
How do manufacturers ensure the quality of silicon epitaxial susceptors?
Manufacturers employ rigorous quality control measures to verify the structural integrity and performance of silicon epitaxial susceptors. Inspection methods, such as microscopic analysis and non-destructive testing, identify defects. Performance tests evaluate thermal conductivity, mechanical strength, and thermal shock resistance.
Are there specific manufacturing methods for silicon epitaxial susceptors?
Yes, manufacturers use specialized methods to produce silicon epitaxial susceptors. These methods include precise shaping, advanced machining techniques, and meticulous surface preparation. Each step ensures the susceptor meets the stringent requirements of semiconductor manufacturing.
What makes silicon carbide a preferred material for susceptors?
Silicon carbide (SiC) is highly valued for its exceptional thermal conductivity and chemical resistance. It withstands extreme temperatures and reduces contamination risks during the epitaxial growth process. These properties make SiC-coated susceptors ideal for producing high-quality semiconductor wafers.
How do silicon epitaxial susceptors contribute to renewable energy?
Silicon epitaxial susceptors are critical in the production of high-efficiency solar cells. These cells rely on defect-free silicon wafers, which are made possible by the precise thermal environment provided by the susceptors. This contribution supports the global shift toward renewable energy solutions.
What industries benefit most from silicon epitaxial susceptors?
Industries such as automotive, aerospace, telecommunications, and renewable energy benefit significantly from silicon epitaxial susceptors. These components enable the production of advanced semiconductor devices, supporting innovations in electric vehicles, autonomous systems, and next-generation communication technologies.