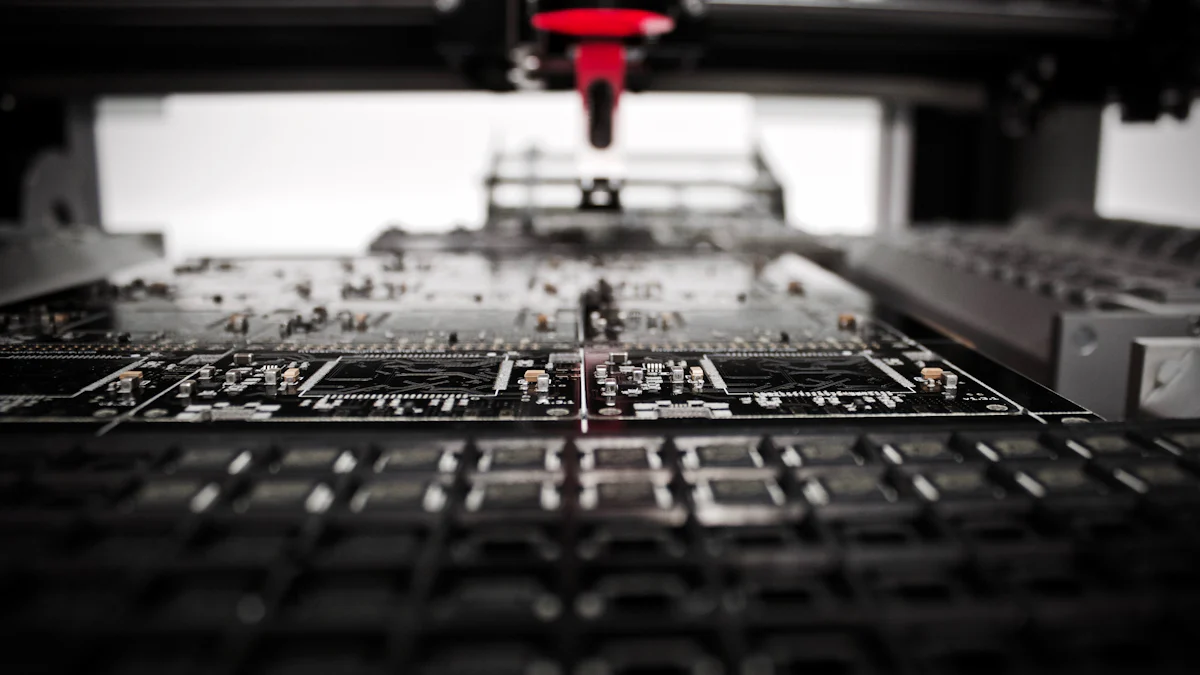
エピタキシーは、基板上の高品質の結晶層の生成を可能にする画期的な結晶成長技術として機能します。 このプロセスは、ピボタルの役割を果たす applied materials epitaxy、材料の特性上の精密な制御を達成することを可能にします。 層によって構造層を精製することによって、, 応用材料のepitaxyの皿 半導体デバイスや先進材料の性能を向上 半導体、ナノテクノロジー、光電子工学などの産業は、大きく依存しています 応用材料 表 イノベーションの境界線を押します。 マイクロチップの開発や通信システム開発のため、, 応用材料 表のプライム 現代の技術に欠かせないまま.
要点
- エピタキシーは、高度な半導体デバイスに不可欠、高品質の結晶層の創造を可能にする精密な結晶成長技術です.
- 均質とヘテロピタキシの違いを理解することは重要です。元は基質と層の同じ材料を使用し、後者は異なる材料を組み合わせて機能を強化します.
- 分子ビームエピタキシー(MBE)、化学蒸気蒸着(CVD)、原子層エピタキシー(ALE)などの主要な方法は、研究から大規模生産まで、特定の用途に固有の利点を提供します.
- エピタキシーは大幅に改善します マイクロチップの性能、LEDsおよび精密な指定に会う欠陥なし、良質材料を保障することによって太陽電池.
- エピタキシプロセスにおけるAIと機械学習の統合は、材料の成長を最適化し、欠陥を削減し、全体的な効率性を向上させるために設定されます.
- エピタキシーは、ナノテクノロジー、光電子工学、再生可能エネルギーなどの分野における先進的な技術で、イノベーションと効率性を促進しています.
- 高いコストと技術的な複雑さにもかかわらず、優れた材料を製造するエピタキシの利点は、最先端のアプリケーションでの使用を正当化します.
応用材料の Epitaxy の理解
What Is Epitaxy?
エピタキシーとは 特殊な結晶成長プロセス 新しい結晶層が注文された方法で基質に形成されるところ。 この技術は、特に半導体や先進材料の開発において、材料科学において重要な役割を果たしています。 エピタキシーを使用することで、高機能電子機器を作るために不可欠である成長した層の結晶性とオリエンテーションを正確に制御することができます.
エピタキシの重要性は、比類のない精度で材料特性を仕立てる能力にあります。 それはあなたがすることができます 工学電気、光学原子レベルの機械的特性。 この制御のレベルは材料がマイクロ チップ、LEDsおよび光電子工学装置のような現代適用のための厳密な条件を満たすことを保障します。 エピタキシーは材料の品質を高めるだけでなく、高度な機能を備えた多機能デバイスの作成を可能にします.
Types of Epitaxy
ホモエピタキシー: 同じ材料の基質上の結晶層の成長
Homoepitaxyは同じ材料の構成を共有する基質で結晶のフィルムを育てることを含みます。 例えば、シリコンウエハにシリコン層を堆積させると、このタイプのエピタキシが生成されます。 半導体製造では、表面品質を磨き、デバイスの性能を改善するために広く使用されています。 素材の均一性を維持することにより、均質化により、シームレスな統合と優れた電気伝導性を保証します.
Heteroepitaxy:異なった材料の基質の結晶の層の成長
一方、ヘテロピタキシーは、異なる材料で構成された基質に結晶層を成長させることを含みます。 一般的な例は、シリコン基板にガリウムアルセニド(GaAs)の堆積物です。 このアプローチは、高度な電子および光電子デバイスを作成するために不可欠です。 Heteroepitaxy は、ナノテクノロジーとフォトニクスの革新のための方法を残す、さまざまな素材のユニークな特性を組み合わせることを可能にします.
均質とヘテロエピタキシーの違い
均質とヘテロピタキシーの間の第一次差別は、基質と成長層の材料組成物にあります。 Homoepitaxyは均一性および両立性に焦点を合わせ、高い純度および欠陥なしの表面を要求する適用にとって理想的にします。 Heteroepitaxy, しかし, 異なる特性を持つ材料を統合することにより、汎用性を強調. この柔軟性により、デバイスを機能とパフォーマンスを向上させることができます.
両方のタイプのエピタキシーは、適用される材料エピタキシーに不可欠です。 薄膜や多層構造を成し遂げ、材料特性を精密に制御できる基盤を提供します。 クリスタルの品質を向上させ、革新的な技術を開発することを目指している場合でも、エピタキシーは現代のマテリアルサイエンスの礎を残します.
エピタキシーテクニックの仕組み
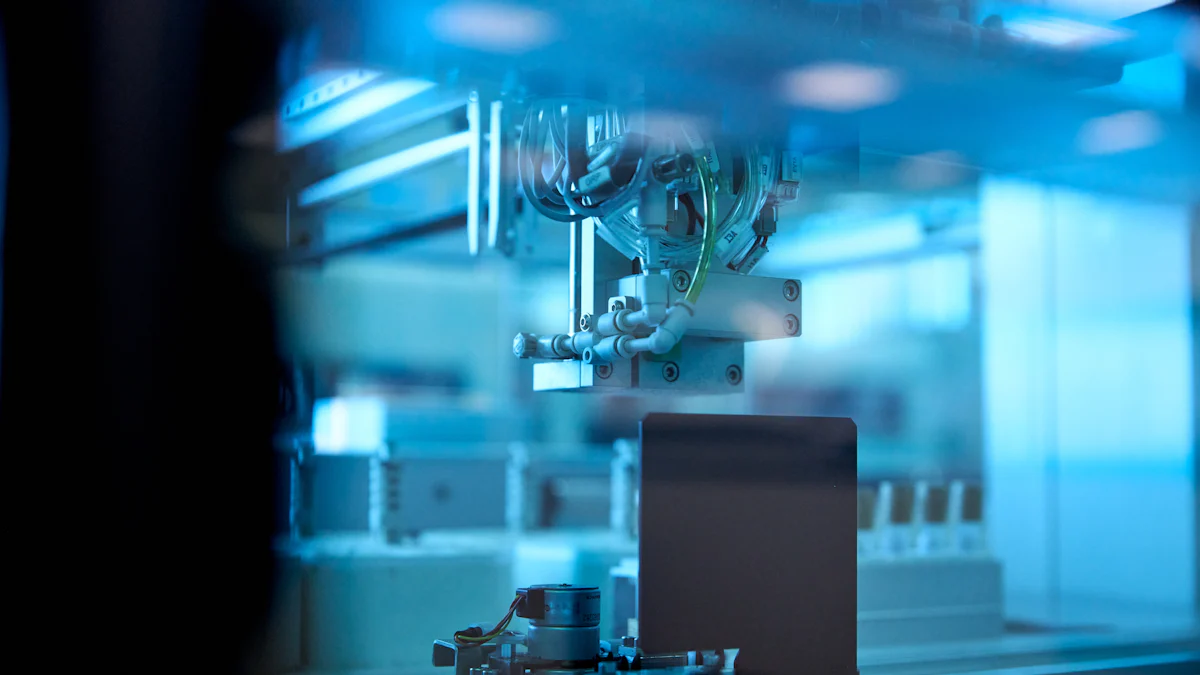
エピタキシープロセスの概要
基板上の薄膜蒸着の解説
エピタキシーは薄膜蒸着で始まり、基質に結晶層を成長させるプロセスです。 この層は注文された方法で形成され、結晶構造が根本的な材料と整列することを確認します。 薄膜蒸着は、精密な電気および光学特性で高品質の材料を作成するために不可欠です。 蒸着環境を慎重に制御することにより、半導体や光電子工学などの高度なアプリケーションにとって重要な均一性と欠陥を減らすことができます.
成長中の結晶配列の維持の重要性
エピタキシャル成長中の結晶の直線を維持すると、材料の構造的完全性が保証されます。 最終製品の性能を損なう欠陥につながる可能性がございます。 このアライメントを維持するために、温度、圧力、および堆積率などの要因を監視する必要があります。 これらの変数は直接結晶の層の質に影響を与えます。 適切なアライメントは、導電性、光明度、および全体的な材料性能を高め、それを応用材料のエピタキシの角質にします.
応用材料の主要方法 Epitaxy
分子ビーム Epitaxy (MBE): 高度に制御された真空ベースのプロセス
分子ビームエピタキシー(MBE)は、超高真空環境を使用して原子層の材料を堆積させます。 この方法では、分子ビームを生成し、基板と相互作用する熱源材料です。 MBEは層の厚さおよび構成上の精密な制御を可能にします。 この技術は、低エネルギー状態の結晶形成を可能にすることにより、不要な欠陥を最小限に抑えます。 MBEは量子の井戸および過格子のような極度な精密を要求する研究開発そして適用にとって理想的です.
化学蒸気の沈殿物(CVD): 化学反応による堆積物への利用方法
化学蒸気蒸着(CVD)は、基質に薄膜を堆積させる化学反応に依存します。 このプロセスでは、ガス前駆体を反応室に導入し、固形層を形成します。 CVDは半導体製造に多様で広く使用されています。 同等体および異方体層の増殖をサポートします。 この方法は、パワーデバイスやLEDに不可欠である炭化ケイ素や窒化ガリウムなどの材料の製造に特に効果的です.
原子層エピタキシー(販売): 超薄層蒸着技術
原子層エピタキシー(Atomic Layer Epitaxy)は、原子レベルの精度で極めて薄い層を堆積させることに焦点を当てています。 この方法は、化学反応を変化させ、各層が一度に1つの原子層を形成することを確認します。 優れた均一性で欠陥のない材料を作成するために、ALEは非常に効果的です。 量子ドットやナノワイヤーなどの高度なナノ構造を開発するために、この技術を使うことができます。 ナノテクノロジーや光電子工学の用途に、その精度が評価可能です.
精密および適用の観点からMBE、CVDおよびALEの比較
各エピタキシメソッドは、ユニークな利点を提供します
- MBEについて バンドギャップ工学のような研究そして専門にされた適用のために適したようにする比類のない精密を提供します.
- CVD スケーラビリティと汎用性に優れ、業界規模の半導体製造に適した製品です.
- アクセサリー 原子レベルの精度、ナノ構造や超薄型フィルムの制作に最適です.
これらの方法を理解することで、特定のニーズに最適な技術を選択できます。 精密、スケーラビリティ、材料の均等性を優先するかどうか、これらの方法は、応用材料のエピタキシーのバックボーンを形成します.
応用材料のエピタキシーの適用
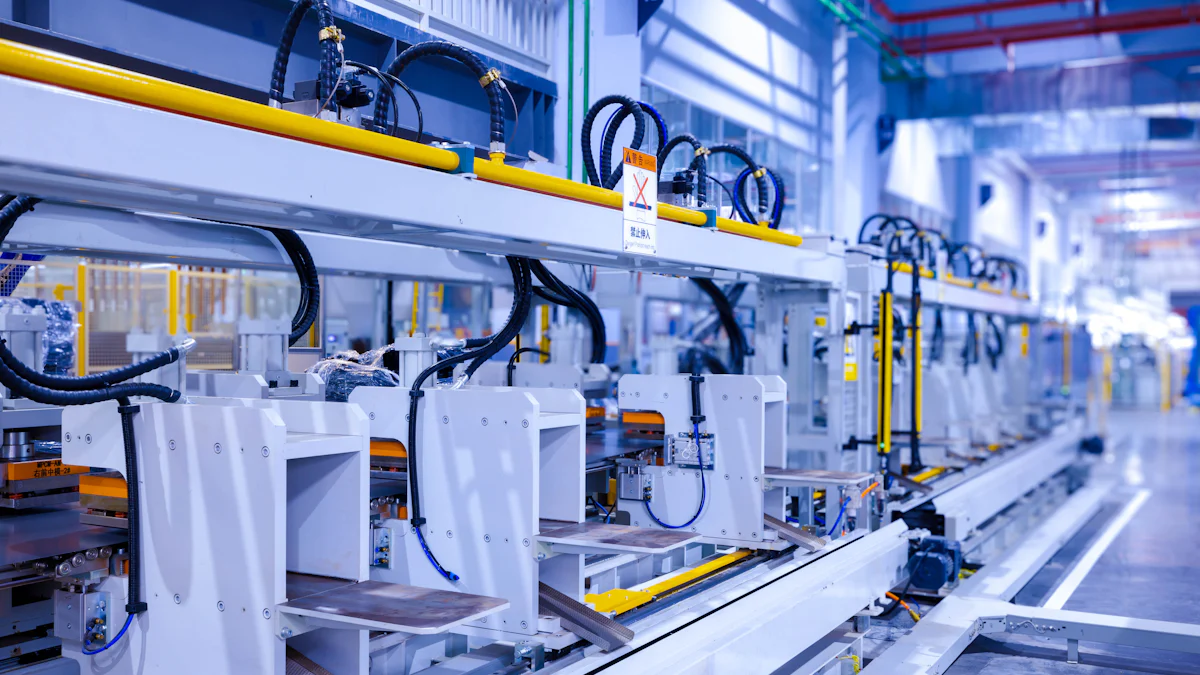
半導体製造
マイクロチップ、トランジスタ、集積回路の作成
エピタキシーは、 生産における重要な役割 マイクロチップ、トランジスタ、集積回路。 高品質の結晶層の成長を可能にすることで、これらのコンポーネントに必要な精度を実現できます。 エピタキシャル プロセスは材料の電気特性が高度の半導体装置のために必要な厳密な指定に合うことを保障します。 この精度は、近代的なコンピューティングと通信技術に不可欠であるより速く、より小さく、より効率的な電子部品を作成することができます.
高効率LEDやレーザーダイオードの製造における役割
照明業界は、エピタキシャル技術から非常に恩恵を受けています。 エピタキシャルウエファーは、高効率LEDやレーザーダイオードの製造に最適な材料を提供します。 これらのデバイスは、エピタキシーが提供する優れた結晶品質と欠陥のない層に依存しています。 エピタキシーを使用して、LEDの明るさ、エネルギー効率および寿命を高めることができます。 レーザダイオードは、光学通信、医療機器、産業機器の用途に理想的な性能を向上しました。 エピタキシーによる材料特性を調整する能力は、これらの装置がさまざまな産業の成長した要求を満たしていることを確認します.
先端材料・技術
量子ドットやナノワイヤーなどのナノテクノロジーの応用
エピタキシーはナノテクノロジーの分野において角質になりました。 量子ドットとナノワイヤの精密な加工を可能にし、次世代技術の開発に不可欠です。 エピタキシャル成長によって作成される量子の点は、例外的な光学および電子特性を提供します。 これらの特性は、ディスプレイ、イメージングシステム、および太陽電池において価値のあるものにします。 同様に、ナノワイヤは、エピタキシーが提供する原子レベルの制御から恩恵を受けており、センサー、トランジスタ、エネルギーストレージデバイス用のユニークな機能を持つ材料を設計することができます.
高度な通信システムのための光電子工学の使用
光検出器や変調器などの光電子デバイスは、その性能のためにエピタキシに依存します。 ヘテロエピタキシャル層を成長させる能力は、材料と異なる特性を組み合わせ、高度な通信システム用に最適化されたデバイスを作成することができます。 エピタキシーはこれらの装置が高い感受性、速度および信頼性を達成することを保障します。 ファイバネットワーク、衛星通信、高速データ伝送で欠かせません。 エピタキシャル技術を活用することで、光電子イノベーションの境界線をプッシュすることができます.
太陽電池などの再生可能エネルギー技術の可能性
再生可能エネルギー技術は、エピタキシーによる大きな進歩を見てきました。 特に、太陽電池は、 高品質の薄膜 エピタキシャルのプロセスによって作り出される。 これらのフィルムは、光吸収とエネルギー変換の効率性を向上させ、より効果的な太陽光発電装置を作成することができます。 エピタキシーはまた、異なる材料の層を積み重ねることで、より高い効率性を達成するマルチジャンクション太陽電池の開発をサポートしています。 この技術は、持続可能なエネルギーソリューションの需要が高まっています.
Advantages and Challenges of Epitaxy
エピタキシーの利点
物質的な成長の高精度および特性上の制御
エピタキシーは他に類を見ない 材料成長の精密、原子レベルで結晶層の特性を制御することを可能にします。 この精密は材料が半導体および光電子工学のような高度の適用のために必要である厳密な指定に会うことを保障します。 エピタキシーを使用することで、電気的、光学的、機械的特性を調整して、特定のニーズに合わせることができます。 この制御レベルは、高性能デバイスを作成するために不可欠であるように、他の材料成長技術とは別にエピタキシを設定します.
「Epitaxyは、優れた純度と完全性でモノクリスタルフィルムの成長を可能にし、最適なデバイス性能を保証します。 ツイート
そのような精度でレイヤーを成長させる能力は、多機能デバイスを設計することができます。 たとえば、さまざまな材料特性を組み合わせるマルチレベル構造を作成し、デバイスの全体的な効率と機能を強化することができます。 この機能は、ナノテクノロジーや再生可能エネルギーなどの業界において特に価値があり、精度はイノベーションに直接影響を与えます.
欠陥のない、良質材料を作成する機能
欠陥のない、良質の結晶の層の作成のEpitaxyのexcels。 プロセスは不純物および構造欠陥を、他の物質的な成長方法の共通の挑戦最小にします。 温度、圧力、ガスの流れなどの要因を慎重に制御することにより、完璧な結晶構造を達成することができます。 これにより、材料は、要求の厳しいアプリケーションでも、一貫した性能を発揮します.
エピタキシーによる高品質レイヤーは、デバイスの耐久性と信頼性を向上させます。 例えば、半導体製造では、欠陥のない層は電気伝導性を高め、エネルギー損失を削減します。 これは、効率的なマイクロチップ、LED、太陽電池を作成するための角質石を作ります。 そのようなものを生成する能力 high-quality materials 最先端の技術を開発する競争上の優位性を与えます.
エピタキシーの挑戦
装置およびプロセスの高コスト
エピタキシーは、特殊な装置と高度に制御された環境を必要とし、生産コストを大幅に増加させます。 超高真空システムの必要性、精密な温度制御および高度の監視用具は最初の投資を実質的にさせます。 多くのメーカーにとって、この高コストは大きなスケールでエピタキシャル技術を採用する障壁を貫く.
費用にもかかわらず, 利点は、多くの場合、コストを上回る. エピタキシーによる高品質の材料と強化されたデバイス性能は、イノベーションに焦点を当てた業界への投資を正当化します。 しかし、生産目標とエピタキシーが整列するかどうかを判断するために、コストの利益率を慎重に評価しなければなりません.
技術的複雑性と専門知識の必要性
エピタキシーなプロセスは、高度な専門知識を要求する複雑な技術的要件を含みます。 ガスの流れ、堆積率、結晶のアライメントなどの複数のパラメータを監視し、制御する必要があります。 未成年の逸脱であっても、最終製品の品質を損なう可能性がある.
「温度、圧力、欠陥生成は、エピタキシャルプロセスにおける正確な制御を必要とする重要な要因です。 ツイート
この複雑さにより、エピタキシーなチャレンジングなテクニックをマスターします。 プロセスのニュアンスを理解し、問題を効果的にトラブルシューティングできる熟練した専門家が必要です。 そのような専門知識のトレーニングと保持は、運用上の課題に追加します。 しかし、高度な自動化とプロセス最適化に投資することで、これらの複雑さの一部を緩和し、効率性を向上させることができます.
応用材料の未来 Epitaxy
エピタキシー技術の革新
コストを削減し、スケーラビリティを改善するためのエマージ方法
応用材料の将来は、コストとスケーラビリティの課題を克服する能力にあります。 研究者やメーカーは、エピタキシーなプロセスを合理化するための革新的な方法を探しています。 例えば、, エピタキシャル機器の進歩 より効率的な材料堆積を可能にし、廃棄物や運用コストを削減します。 これらの改善は、禁止コストを調達することなく、エピタキシー技術を採用することができます.
エコノミも、エピタキシー市場で成長しています。 日本のような国は、電子機器の需要が高まっているため、伝染技術に大きく投資しています。 このグローバル展開は、コスト効率の高いソリューションの開発を促す競争を促進します。 これらの新しい方法を採用することにより、高度なアプリケーションに必要な高品質の基準を維持しながら、生産をスケールアップすることができます.
プロセス最適化のためのAIと機械学習との統合
人工知能(AI)と機械学習(ML)は、エピタキシーなプロセスに革命をもたらします。 これらの技術は、材料のあらゆる段階の成長を監視し、最適化することができます。 たとえば、AIアルゴリズムはリアルタイムデータを分析し、温度やガスの流れなどのパラメータを調整し、一貫性のある結晶品質を保証します。 この精度は欠陥を最小化し、プロセスの全体的な効率を高めます.
機械学習モデルは、潜在的な問題が発生した前にも予測します。 歴史データにおけるパターンを識別することにより、MLツールは、あなたの技術とダウンタイムを削減するのに役立ちます。 AIとMLの統合は、エピタキシの信頼性を向上させるだけでなく、イノベーションを加速します。 これらの技術は、材料科学において可能なものの境界線をプッシュすることを可能にします.
エピタキシーのアプリケーションを拡大
量子コンピューティングと次世代エレクトロニクスの推進
エピタキシーは量子コンピューティングと次世代エレクトロニクスの画期的な方法を舗装しています。 素材特性の正確な制御により、量子デバイスに必要な量子ドットやその他のナノ構造を作成できます。 これらの構造は、ユニークな電子行動を展示し、量子ビット(量子ビット)や他の高度なコンポーネントに最適です.
次世代エレクトロニクスでは、高性能トランジスタや集積回路の開発をサポートしています。 例えば、Nitride Epitaxy は、電力と無線周波数のトランジスタを強化するために使用されます。 これらの進歩により、近代的な技術の要求を満たすより速く、より効率的なデバイスの設計が可能になります。 エピタキシーを活用することで、コンピューティングと電子システムの進化に貢献できます.
医療機器やセンサーの遮断の可能性
医療分野は、エピタキシーの進歩からも恩恵を受けています。 高品質のエピタキシャルレイヤーにより、感度と精度の優れたセンサーを開発できます。 これらのセンサーは、診断イメージングや患者モニタリングなどの用途に不可欠です。 素材特性を調整する能力は、これらのデバイスがさまざまな条件下で確実に実行できるようにします.
エピタキシーは、インプラント可能な医療機器のイノベーションを推進しています。 例えば、生体適合性材料の精密な成長により、人体組織とシームレスに統合するデバイスを作成することができます。 患者の成果を改善し、医療技術の新たな可能性を広げます。 エピタキシーに焦点を合わせることで、ヘルスケアソリューションの高度化に重要な役割を果たします.
エピタキシーは応用材料のエピタキシーのコーナーストーンとして、先端技術の精密な工学を可能にします。 素材の特性を磨き、クリスタル品質を向上することで、半導体、ナノテクノロジー、光エレクトロニクスなどの業界向け高性能デバイスを作成することができます。 この技術は、多機能デバイスの開発だけでなく、5G、IoT、再生可能エネルギーなどの次世代技術のイノベーションを推進しています。 進歩が続くにつれて、エピタクシーは近代的な技術の未来を形作るために巨大な潜在能力を保持し、画期的なアプリケーションのための無限の可能性を提供します.
よくあるご質問
What is epitaxy?
エピタキシーは、加工された単結晶基材に新しい単結晶層を成長させるプロセスを指します 炭化ケイ素(SiC)基質. . 基質(homoepitaxy)の材料と一致するか、またはそれ(heteroepitaxy)と異なってもよい。 エピタキシャル層として知られる新しく成長させた層は、基質の水晶構造と一直線に合わせます。 通常、この層は厚さのマイクロメートルだけを測定します。 例えば、シリコン系エピタキシーでは、特定のオリエンテーション、抵抗性、SiC基質の厚さで単結晶シリコン層を育てます。 結合すると、基質およびエピタキシャル層はエピタキシャルウエファーと呼ばれるものを形成します.
半導体製造におけるエピタキシ(epi)プロセスとは?
半導体製造におけるエピタキシーなプロセスは、特定の方向に結晶基質の上に薄結晶層を成長させることを含みます。 このステップは、集積回路や半導体デバイスを構築するための完璧な結晶基盤を作成するために不可欠です。 プロセスは単一の水晶基質で厚い0.5から20ミクロン間の単一の水晶材料の微細な層を、普通預金します。 この技術は、特にシリコンウェーハ製造において、半導体デバイスの製造において重要な役割を果たし、高度な用途に高品質の材料を確保しています.
エピタキシーが半導体デバイスの性能を改善するには?
エピタキシーは、欠陥のない高品質の結晶層を提供することで、半導体デバイス性能を向上させます。 この層は、マイクロチップ、LED、トランジスタなどの高度なデバイスに不可欠である電気、光学、および機械的特性上の正確な制御を保証します。 材料特性を原子レベルで調整することにより、エピタキシーは、デバイスにおける優れた導電性、効率性、信頼性を実現します.
What are the main types of epitaxy?
エピタキシの2つの主要なタイプは次のとおりです
- Homoepitaxy: : : 同じ材料の基質に結晶層を成長させる。 例えば、シリコンウェーハにシリコンを蒸着することで、均一性と互換性が確保されます.
- Heteroepitaxy: : : これは、異なる材料の基質に結晶層を成長させることを含みます。 シリコン基板にガリウムアルセニド(GaAs)を堆積させる例で、両方の材料のユニークな特性を兼ね備えています.
各タイプは、目的のアプリケーションや素材の特性に応じて、特定の目的に役立ちます.
エピタキシーで重要な結晶配列はなぜですか?
結晶アライメントは、エピタキシャル層の構造的完全性と性能を保証します。 最終製品の品質と機能性を損なう欠陥につながる可能性がございます。 成長中の適切なアライメントを維持することで、材料の導電性、光明度、および全体的な性能を高めます。 温度、圧力、および沈殿率のような要因はこの直線を達成するために慎重に制御されなければなりません.
エピタキシで使用される重要な方法は?
主な方法は次のとおりです
- Molecular Beam Epitaxy (MBE): : : 層の厚さおよび構成上の精密な制御を提供する真空ベースのプロセス.
- 化学気相成長法(CVD): : : 薄膜を堆積させるための化学反応を用いる汎用性の高い方法.
- 原子層エピタキシー(販売): : : 原子レベルの精度で超薄型層を堆積させる技術.
各方法は、研究から大規模生産まで、さまざまなアプリケーションに適したユニークな利点を持っています.
再生可能エネルギー技術のエピタキシーはどのように貢献しますか?
エピタキシーは、特に太陽電池開発において、再生可能エネルギーの重要な役割を果たしています。 高品質のエピタキシャル層は、光吸収とエネルギー変換効率を向上させます。 複数の接合太陽電池、エピタキシー、異なる材料のスタック層によって作成され、より高い効率を実現します。 持続可能なエネルギーソリューションの需要が高まっています.
What industries benefit the most from epitaxy?
半導体、ナノテクノロジー、光電子工学、再生可能エネルギーなどの産業は、エピタキシーで有意に利益をもたらします。 マイクロチップ、LED、量子ドット、太陽電池などの高度なデバイスの作成が可能です。 精密な特性を持つ高品質の材料を提供することにより、エピタキシーは、これらの分野におけるイノベーションを推進しています.
大量生産にエピタキシをスケールアップできますか?
はい、エピタキシャル装置および技術の進歩は大量生産のためのepitaxyを拡張することを可能にします。 化学蒸気蒸着(CVD)のような方法は、工業スケールの製造に特に有効です。 エマージ技術は、コストを削減し、スケーラビリティを向上させることを目指し、大規模アプリケーション向けによりアクセス可能なエピタキシーを実現します.
エピタキシの未来とは?
エピタキシーの未来は、コストを削減し、スケーラビリティを改善し、AIや機械学習などの高度な技術を統合するイノベーションにあります。 これらの進歩は、エピタキシプロセスを最適化し、材料の品質を高め、量子コンピューティング、医療機器、次世代エレクトロニクスなどの分野におけるアプリケーションを拡大します。 エピタキシーは、現代技術の未来を形作り続けます.