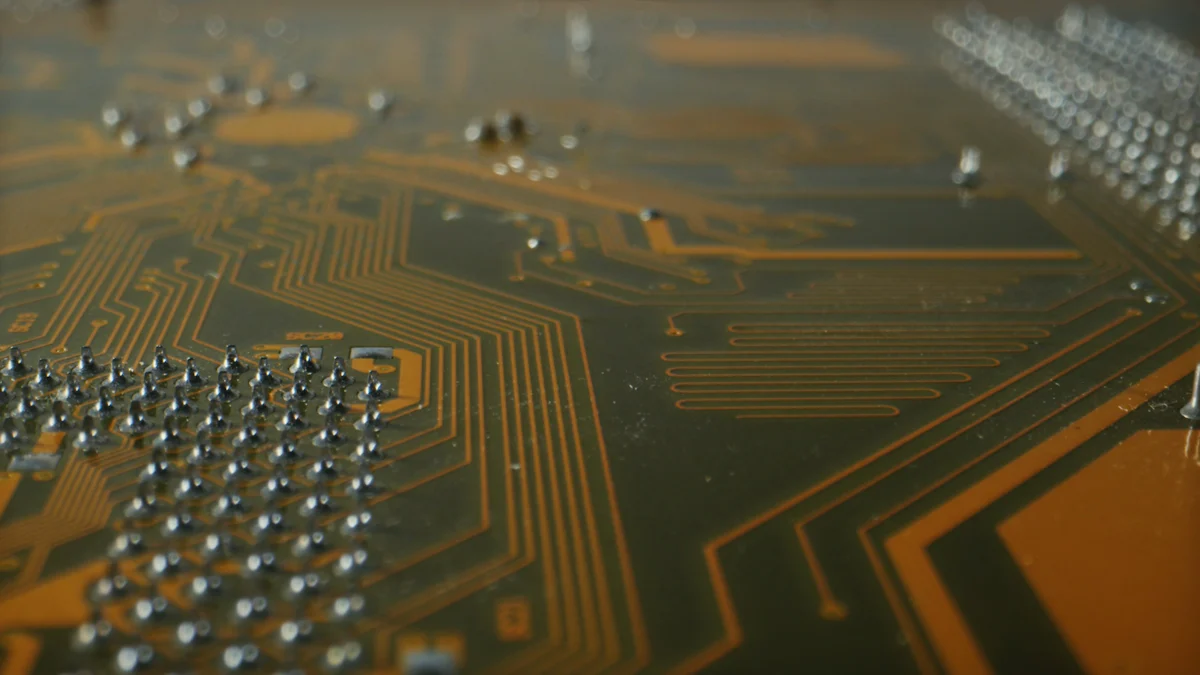
Epitaxy serves as a cornerstone in modern electronics, enabling the creation of crystalline layers with unmatched precision. This process enhances the structural and electrical properties of semiconductor materials, making them indispensable for advanced technologies. By tailoring crystal growth, epitaxy improves device performance in applications of epitaxy ranging from microprocessors to optoelectronics. The global epitaxy equipment market, valued at 4.42 billion USD in 2023, highlights its growing significance. Companies like Applied Materials epitaxy contribute to this evolution by offering cutting-edge applied materials semiconductor equipment. These innovations drive the development of applied materials products, ensuring the seamless integration of epitaxy into next-generation devices.
Key Takeaways
- Epitaxy is essential for creating high-quality crystalline layers, enhancing the performance of semiconductor devices used in technologies like 5G and AI.
- Molecular-beam epitaxy (MBE) allows for atomic-level control of layer thickness, crucial for developing advanced semiconductor structures.
- Remote epitaxy enables the reuse of substrates, reducing costs and improving material efficiency, making it ideal for flexible electronics.
- Van der Waals epitaxy broadens the range of materials that can be integrated into devices, eliminating the need for lattice matching.
- Selective Area Epitaxy (SAE) offers precise control over growth rates, essential for producing specialized structures in semiconductor lasers and photonic circuits.
- Epitaxy is driving innovations in emerging technologies, including quantum computing and flexible electronics, by enabling the fabrication of high-performance materials.
- Despite its advantages, epitaxy faces challenges in scalability and technical precision, necessitating ongoing research to enhance its manufacturing processes.
Epitaxial Growth Methods and Their Features
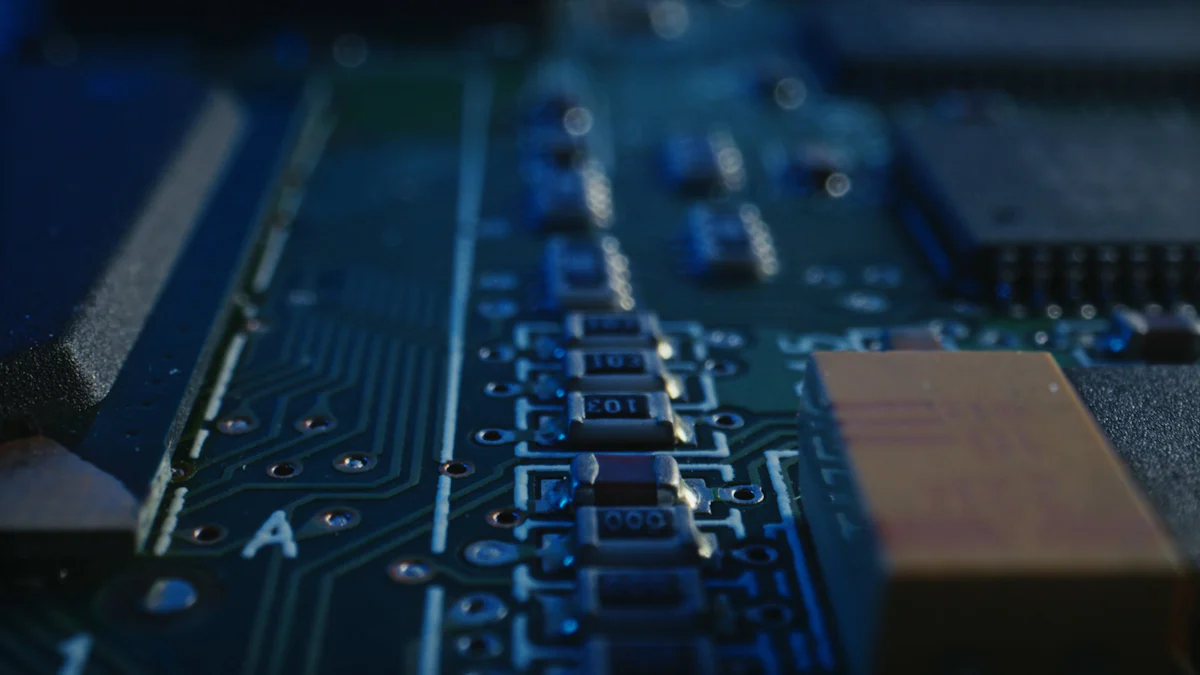
Molecular-Beam Epitaxy (MBE)
Molecular-beam epitaxy (MBE) stands out as a highly precise technique for growing thin crystalline layers. This method involves directing molecular or atomic beams onto a heated substrate under ultra-high vacuum conditions. The controlled environment ensures the deposition of high-purity films with minimal defects. MBE is widely used for fabricating semiconducting compounds like silicon, germanium, and gallium arsenide.
Key Feature: MBE allows researchers to manipulate layer thickness at the atomic level, enabling the creation of complex structures for advanced semiconductor devices.
The versatility of MBE makes it essential for developing quantum wells, superlattices, and other nanostructures. Its precision supports innovations in optoelectronics and high-speed transistors.
Remote Epitaxy
Remote epitaxy introduces a groundbreaking approach by leveraging a graphene interlayer between the substrate and the epitaxial film. This technique enables the growth of high-quality crystalline layers while maintaining the ability to peel off the film for reuse. The process enhances material efficiency and reduces production costs.
Scientific Research Findings: Studies have demonstrated that remote epitaxy facilitates the creation of freestanding single-crystalline nanomembranes. These membranes find applications in flexible electronics and wearable devices.
This method also supports the growth of various materials, including compound semiconductors and oxides. Its adaptability positions it as a key player in next-generation technologies.
Van der Waals Epitaxy
Van der Waals epitaxy (vdW epitaxy) employs weak van der Waals forces to grow crystalline films on substrates with mismatched lattice structures. This flexibility allows the deposition of materials that would otherwise be incompatible. For instance, researchers have successfully grown β-Ga2O3 films on graphene/SiC substrates using vdW epitaxy.
Key Advantage: The process eliminates the need for lattice matching, broadening the range of materials that can be integrated into electronic devices.
Flexible electronics benefit significantly from vdW epitaxy due to its ability to produce freestanding epitaxial films. This method also supports the development of advanced optoelectronic devices and high-performance transistors.
These epitaxial growth methods showcase the diverse approaches to tailoring crystalline layers for modern electronics. Each technique offers unique advantages, driving innovation across semiconductor, optoelectronic, and emerging technology applications.
Selective Area Epitaxy (SAE)
Selective Area Epitaxy (SAE) represents a pivotal technique in modern semiconductor fabrication. This method enables the localized growth of crystalline layers by masking specific regions of a substrate. Engineers use this precision to create intricate structures essential for advanced electronic and photonic devices.
Key Advantage: SAE allows for precise control over growth rates and material composition, enabling the development of devices with tailored electrical and optical properties.
Applications in Semiconductor Lasers
SAE plays a critical role in the production of semiconductor lasers. It facilitates the fabrication of buried heterojunction (BH) lasers, which are known for their high efficiency and stability. Additionally, SAE supports the creation of quantum dot (QD) lasers, which offer superior performance in terms of wavelength tunability and energy efficiency. These lasers find applications in telecommunications, medical devices, and high-speed data transmission.
Photonic Integrated Circuits (PICs)
The versatility of SAE extends to photonic integrated circuits (PICs). Engineers utilize this technique to develop compact devices such as electro-absorption modulated lasers (EMLs) and multi-wavelength array lasers (MWLAs). These components are integral to modern optical communication systems, enabling faster and more reliable data transfer.
Scientific Research Findings: Studies highlight SAE’s ability to integrate III–V compound lasers on silicon substrates. This advancement bridges the gap between traditional silicon-based electronics and high-performance photonic devices.
Bandgap Engineering and Composition Control
SAE also excels in bandgap engineering, a process that adjusts the energy levels of materials to optimize their electronic and optical behavior. By controlling the composition of the grown layers, SAE enables the creation of devices with enhanced functionality. For instance, it supports the development of heteroepitaxial lasers on silicon, which combine the benefits of silicon’s scalability with the superior performance of III–V semiconductors.
Benefits of SAE in Modern Electronics
- Precision: SAE ensures localized growth, reducing material waste and improving device efficiency.
- Scalability: The technique adapts well to large-scale manufacturing processes, making it suitable for industrial applications.
- Versatility: SAE supports a wide range of materials and device architectures, from lasers to photonic circuits.
Selective Area Epitaxy continues to drive innovation in the semiconductor industry. Its ability to create highly specialized structures positions it as a cornerstone for next-generation technologies, including optical communications and integrated photonics.
Applications of Epitaxy in Modern Electronics
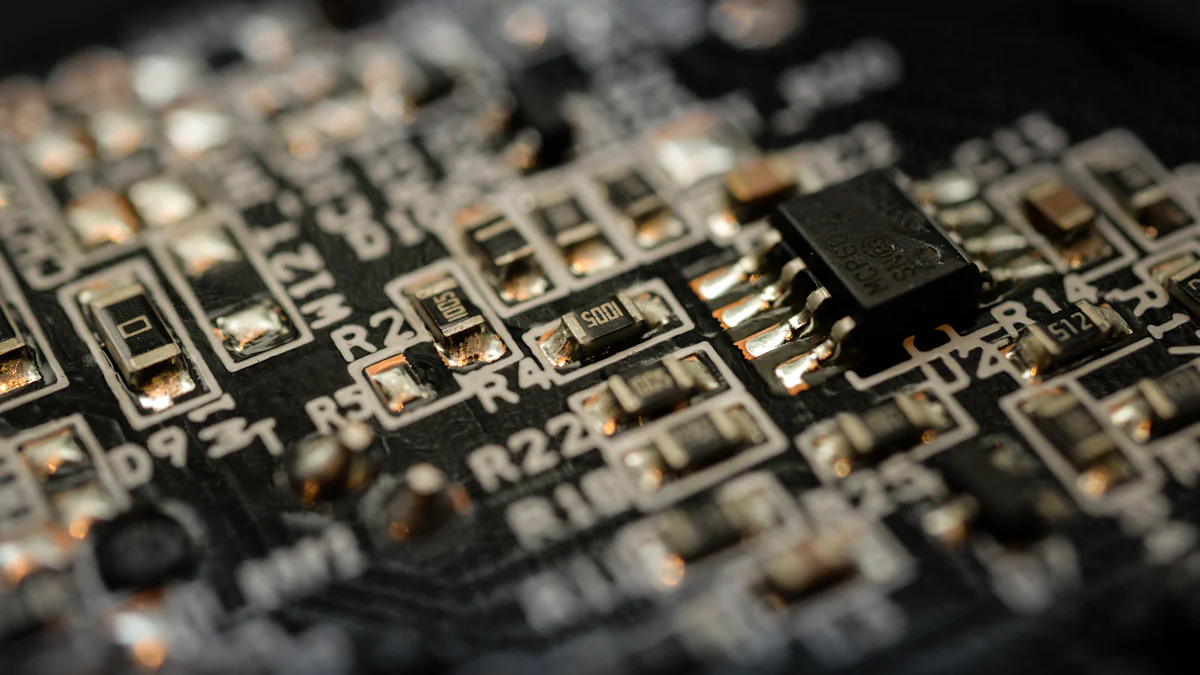
Epitaxy has revolutionized the electronics industry by enabling the precise engineering of crystalline layers. Its ability to enhance material properties has made it indispensable in various fields. Below are some of the most impactful applications of epitaxy in modern electronics.
Semiconductor Devices
Epitaxy plays a pivotal role in the fabrication of advanced semiconductor devices. By creating thin crystalline layers with exceptional purity and uniformity, epitaxy ensures superior electrical performance. This process is critical for manufacturing components such as transistors, diodes, and integrated circuits.
Key Insight: The demand for high-performance semiconductor devices in 5G telecommunications, artificial intelligence (AI), and automotive electronics has driven significant investments in epitaxy equipment.
Epitaxy enables the production of compound semiconductors like gallium arsenide and silicon carbide, which outperform traditional silicon in specific applications. These materials are essential for high-speed communication systems and power electronics. Furthermore, epitaxy enhances the scalability of complementary metal-oxide-semiconductor (CMOS) technology, ensuring its compatibility with next-generation devices.
Optoelectronics
The field of optoelectronics benefits immensely from the applications of epitaxy. This process facilitates the growth of high-quality crystalline layers required for light-emitting diodes (LEDs), laser diodes, and photodetectors. Epitaxial techniques allow precise control over material composition, enabling the development of devices with tailored optical properties.
Example: Epitaxy supports the creation of quantum dot lasers, which offer superior wavelength tunability and energy efficiency. These lasers are vital for telecommunications and medical imaging.
Epitaxy also contributes to the advancement of photonic integrated circuits (PICs). These circuits integrate multiple optical components onto a single chip, reducing size and improving performance. The precision of epitaxial growth ensures the seamless integration of materials like indium phosphide and gallium nitride, which are crucial for high-efficiency optoelectronic devices.
Photovoltaics
In the realm of photovoltaics, epitaxy has emerged as a game-changer. It enables the production of high-efficiency solar cells by creating defect-free crystalline layers. Epitaxial growth techniques allow manufacturers to optimize the bandgap of materials, maximizing their ability to convert sunlight into electricity.
Notable Development: Epitaxy has facilitated the development of multi-junction solar cells, which achieve higher efficiencies by stacking layers with different bandgaps.
These advanced solar cells are widely used in space applications, where efficiency and reliability are paramount. Additionally, epitaxy supports the growth of thin-film solar cells, which are lightweight and flexible. This innovation has expanded the use of solar technology in portable and wearable devices.
The applications of epitaxy in modern electronics underscore its transformative impact. From enhancing semiconductor performance to driving innovations in optoelectronics and photovoltaics, epitaxy continues to shape the future of technology.
Emerging Technologies
Epitaxy continues to drive innovation in emerging technologies, shaping the future of electronics, optics, and quantum physics. Advances in epitaxial materials have unlocked new possibilities for creating devices with unparalleled performance and efficiency. These breakthroughs are paving the way for transformative applications in cutting-edge fields.
Quantum Computing and Quantum Devices
Epitaxy plays a crucial role in the development of quantum computing. The precision of epitaxial growth enables the fabrication of qubits, the fundamental units of quantum information. By creating defect-free crystalline layers, epitaxy ensures the stability and coherence of qubits, which are essential for reliable quantum operations.
Notable Advancement: Researchers have utilized epitaxial techniques to produce high-quality silicon-germanium heterostructures, which serve as a platform for spin-based qubits. These structures enhance the scalability and performance of quantum processors.
Quantum devices, such as single-photon detectors and quantum dot lasers, also benefit from epitaxial growth. These components are vital for quantum communication systems, which promise secure data transmission through quantum encryption.
Flexible and Wearable Electronics
The rise of flexible and wearable electronics has been fueled by innovations in epitaxial materials. Van der Waals epitaxy, in particular, has enabled the creation of freestanding crystalline films that are lightweight and flexible. These films are ideal for applications in bendable displays, smart textiles, and medical sensors.
Key Insight: Flexible electronics rely on epitaxial films to maintain high electrical performance while adapting to various shapes and movements. This capability is transforming industries such as healthcare, where wearable devices monitor vital signs in real time.
Epitaxy also supports the integration of advanced materials like gallium nitride and indium phosphide into flexible platforms. These materials enhance the functionality of devices, enabling features such as high-speed data processing and efficient energy harvesting.
Advanced Optics and Photonics
Epitaxy has revolutionized the field of optics and photonics by enabling the precise engineering of light-manipulating materials. Photonic integrated circuits (PICs), which combine multiple optical components on a single chip, rely heavily on epitaxial growth. These circuits are essential for applications in telecommunications, data centers, and autonomous vehicles.
Scientific Development: Epitaxial techniques have facilitated the creation of multi-wavelength lasers and high-efficiency photodetectors. These advancements improve the speed and reliability of optical communication systems.
In addition, epitaxy supports the development of metasurfaces—ultrathin materials that manipulate light at the nanoscale. These surfaces are being explored for applications in augmented reality (AR) devices, holographic displays, and advanced imaging systems.
High-Performance Energy Solutions
Emerging energy technologies are leveraging the benefits of epitaxy to achieve higher efficiency and reliability. Multi-junction solar cells, which stack epitaxial layers with different bandgaps, have set new records for solar energy conversion. These cells are widely used in space exploration, where energy efficiency is critical.
Epitaxy also contributes to the advancement of solid-state batteries. By creating thin crystalline layers with tailored properties, epitaxy enhances the performance of battery electrodes, leading to longer-lasting and safer energy storage solutions.
The applications of epitaxy in emerging technologies highlight its transformative potential. From quantum computing to flexible electronics and advanced optics, epitaxy continues to push the boundaries of innovation. Its ability to engineer materials at the atomic level ensures its relevance in shaping the technologies of tomorrow.
Challenges and Limitations of Epitaxy
Technical Challenges
Epitaxy demands precise control over the growth process to achieve high-quality crystalline layers. This precision requires advanced equipment and meticulous sample preparation. For instance, maintaining uniform thickness in epitaxial layers is critical for ensuring optimal semiconductor device performance. Any deviation in thickness can lead to defects, which compromise the electrical and optical properties of the material.
Scientific Research Findings: The JXT Wafer Article highlights that controlling the thickness of epitaxial layers remains a significant challenge in producing high-performance wafers. This issue directly impacts the efficiency and reliability of semiconductor devices.
Another technical hurdle involves the integration of different materials with mismatched lattice structures. While techniques like van der Waals epitaxy address this issue, they still face limitations in achieving perfect alignment. Additionally, the process often requires ultra-high vacuum conditions, which increase operational complexity and costs.
The development of freestanding epitaxial films also presents challenges. Methods such as mechanical spalling, used to create these films, often struggle with substrate reuse and interlayer removal. These limitations hinder the scalability and cost-effectiveness of such techniques.
Key Insight: Advanced epitaxy equipment integrates cutting-edge technologies to enhance process control. However, the complexity of these systems necessitates continuous research and development to overcome existing technical barriers.
Scalability Concerns
Scaling epitaxial processes for high-volume manufacturing poses significant challenges. The intricate nature of epitaxial growth, combined with the need for precise control, makes it difficult to adapt these techniques for mass production. Manufacturers must balance quality and efficiency, which often leads to increased production costs.
Market Insights: According to the Market Research Future Report, the epitaxy equipment market is heavily influenced by the growing demand for advanced semiconductor devices. However, meeting this demand requires scalable solutions that maintain the integrity of epitaxial layers.
Substrate reuse is another scalability concern. Techniques like remote epitaxy aim to address this by enabling the detachment and reuse of substrates. Despite these advancements, achieving consistent results across large-scale operations remains a challenge. The high cost of substrates further complicates efforts to scale epitaxial processes.
The production of compound semiconductors, such as gallium arsenide and silicon carbide, also faces scalability issues. These materials require specialized epitaxial techniques, which are often less adaptable to industrial-scale manufacturing compared to traditional silicon-based processes.
Scientific Development: The Nano Convergence Journal Article emphasizes the limitations of mechanical spalling in substrate reuse. Overcoming these limitations is essential for making epitaxial processes more scalable and cost-effective.
Epitaxy continues to face technical and scalability challenges that hinder its widespread adoption in high-volume manufacturing. Addressing these limitations requires ongoing innovation in equipment design, process optimization, and material engineering. By overcoming these obstacles, epitaxy can unlock its full potential in shaping the future of modern electronics.
Future Opportunities and Innovations in Epitaxy
Advancements in Epitaxial Techniques
Epitaxial techniques continue to evolve, offering new possibilities for material engineering and device fabrication. Researchers are developing innovative methods to overcome the limitations of traditional epitaxy. For instance, remote epitaxy introduces a graphene interlayer, enabling the detachment and reuse of substrates. This advancement reduces production costs and enhances material efficiency. Similarly, van der Waals epitaxy eliminates the need for lattice matching, allowing the integration of materials with mismatched structures. These techniques expand the range of materials available for advanced applications.
Scientific Research Findings: Recent studies highlight the potential of emerging epitaxy techniques to produce freestanding nanomembranes. These membranes are lightweight and flexible, making them ideal for wearable electronics and bendable displays.
The integration of advanced epitaxy equipment has also improved process control. Ultra-high vacuum chambers and precise precursor gas delivery systems ensure the growth of high-quality crystalline layers. These systems support the production of single crystals and thin films, which are essential for next-generation semiconductor devices.
Key Insight: The growing demand for 5G and IoT technologies has driven significant investments in epitaxial growth techniques. These advancements enable the precise engineering of semiconductor layers, ensuring superior performance in modern devices.
Expanding Applications in Next-Generation Technologies
Epitaxy is unlocking new opportunities in next-generation technologies, shaping the future of electronics, optics, and quantum physics. In quantum computing, epitaxial growth plays a critical role in fabricating qubits. The precision of this process ensures the stability and coherence of qubits, which are essential for reliable quantum operations. High-quality silicon-germanium heterostructures, produced through epitaxy, enhance the scalability of quantum processors.
Notable Development: Researchers have utilized epitaxial techniques to create single-photon detectors and quantum dot lasers. These components are vital for quantum communication systems, which promise secure data transmission.
Flexible and wearable electronics also benefit from epitaxial innovations. Freestanding crystalline films, produced through van der Waals epitaxy, maintain high electrical performance while adapting to various shapes. These films are transforming industries such as healthcare, where wearable devices monitor vital signs in real time.
In the field of advanced optics, epitaxy supports the development of photonic integrated circuits (PICs). These circuits combine multiple optical components on a single chip, improving the speed and reliability of optical communication systems. Epitaxial techniques also enable the creation of metasurfaces, which manipulate light at the nanoscale. These surfaces are being explored for applications in augmented reality (AR) devices and holographic displays.
Key Insight: Multi-junction solar cells, made possible by epitaxial growth, achieve higher efficiencies by stacking layers with different bandgaps. These cells are widely used in space exploration and renewable energy solutions.
The continuous advancements in epitaxial techniques and their expanding applications demonstrate the transformative potential of this technology. By enabling precise material engineering, epitaxy is paving the way for innovations in quantum computing, flexible electronics, and advanced optics. Its role in shaping next-generation technologies underscores its importance in the future of modern electronics.
Epitaxy has redefined the landscape of modern electronics by enabling the precise engineering of semiconductor layers. This transformative process enhances device performance, paving the way for advancements in technologies like 5G, IoT, and quantum computing. Its ability to synthesize high-quality crystalline materials ensures the development of innovative applications across industries.
Epitaxy not only strengthens current semiconductor technologies but also unlocks possibilities for next-generation devices.
As researchers refine epitaxial techniques, its role in shaping the future of electronics and optoelectronics will continue to expand, driving progress and innovation in an increasingly connected world.
FAQ
What is epitaxy and how is it produced?
Epitaxy refers to a material deposition process where thin crystalline layers, known as epilayers, are grown on a substrate. These layers replicate the crystallographic structure of the substrate, ensuring high precision and uniformity. This process is essential for creating materials with superior electrical and optical properties.
Key Insight: The epitaxial layer mimics the substrate’s crystalline structure, making it indispensable in semiconductor fabrication.
What are the different types of epitaxy?
Epitaxy can be categorized into two main types:
- Homoepitaxy: This involves growing a film on a substrate of the same material. For example, silicon films grown on silicon substrates.
- Heteroepitaxy: This involves growing a film on a substrate with a different composition. An example is gallium arsenide films grown on silicon substrates.
Each type serves specific applications, depending on the desired material properties and device requirements.
How does epitaxy contribute to the semiconductor industry?
Epitaxy plays a pivotal role in the semiconductor industry by enabling the growth of thin crystalline layers during the fabrication process. These layers enhance the performance of semiconductor devices such as transistors, diodes, and integrated circuits.
Example: Epitaxy ensures the production of high-quality materials like gallium arsenide and silicon carbide, which are critical for high-speed communication systems and power electronics.
What are some applications of epitaxy?
Epitaxy finds applications across various fields, including:
- Nanotechnology: Used for creating nanoscale structures with precise control.
- Semiconductor Fabrication: Essential for manufacturing advanced electronic components.
- Surface Science: Enables the study and manipulation of material surfaces.
- Optoelectronics: Supports the development of LEDs, laser diodes, and photodetectors.
Its versatility makes it a cornerstone in modern electronics and emerging technologies.
How is epitaxial growth utilized in producing single crystals and thin films?
Epitaxial growth facilitates the production of single crystals and thin films by depositing layers with exceptional purity and uniformity. This process is widely used in semiconductor research to create materials with tailored properties for specific applications.
Scientific Development: Researchers use epitaxial growth to produce freestanding thin films, which are integral to flexible electronics and wearable devices.
What role does epitaxy equipment play in semiconductor device fabrication?
Epitaxy equipment is vital for depositing thin crystalline layers onto substrates. These layers form the foundation for advanced electronic components, including integrated circuits, LEDs, and photonic devices.
Key Advantage: Modern epitaxy equipment ensures precise control over layer thickness and composition, enabling the production of high-performance devices.
How does epitaxy enhance optoelectronic devices?
Epitaxy enables the precise engineering of materials used in optoelectronic devices. It supports the growth of high-quality layers for light-emitting diodes (LEDs), laser diodes, and photodetectors. These devices benefit from the tailored optical properties achieved through epitaxial techniques.
Example: Quantum dot lasers, developed using epitaxy, offer superior wavelength tunability and energy efficiency, making them essential for telecommunications and medical imaging.
Why is epitaxy important for photovoltaics?
Epitaxy plays a crucial role in photovoltaics by enabling the production of high-efficiency solar cells. It allows manufacturers to create defect-free crystalline layers, optimizing the bandgap of materials for maximum sunlight conversion.
Notable Application: Multi-junction solar cells, made possible by epitaxy, achieve higher efficiencies by stacking layers with different bandgaps. These cells are widely used in space exploration and renewable energy solutions.
How does epitaxy support emerging technologies?
Epitaxy drives innovation in emerging technologies such as quantum computing, flexible electronics, and advanced optics. It enables the creation of materials with unparalleled precision, supporting the development of qubits, freestanding films, and photonic integrated circuits.
Key Insight: Epitaxial techniques are unlocking new possibilities in fields like augmented reality, wearable devices, and quantum communication systems.
What challenges does epitaxy face in large-scale manufacturing?
Scaling epitaxial processes for high-volume production presents challenges. Maintaining uniformity and quality across large substrates requires advanced equipment and meticulous process control. Substrate reuse and cost-effectiveness also remain areas of focus for researchers and manufacturers.
Scientific Research Findings: Innovations like remote epitaxy aim to address these challenges by enabling substrate detachment and reuse, reducing costs while maintaining material efficiency.